ATP synthesis
PDFATP plays a central role in cellular metabolism. [1] It is the most important donor of free energy in any biological system. In eukaryotes, its synthesis takes place in the mitochondria – during respiration – and in the chloroplasts – during photosynthesis. It is this last example that is taken here.
Within the thylacoid membrane (see Shedding light on photosynthesis), light induces electron transfer from water to NADP+ through photosystems II and I, the cytochrome b6f complex, and through various mobile molecules: plastoquinones, plastocyanin and ferredoxin (Figure 1) [2]. The flow of electrons along this membrane chain generates a proton concentration gradient, and thus an electrochemical gradient, across the thylakoid membrane. This gradient is used by ATP synthase for the phosphorylation of ADP to ATP. [3]
1. Establishing a transmembrane pH gradient
1.1. Protons and linear electron transfer
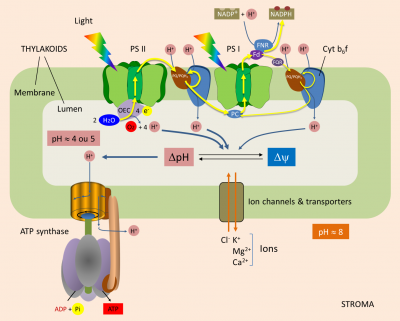
Water oxidation. Each photon reaching the P680 (at the PSII) induces a charge separation that initiates the transfer of electrons to the PSI and NADP+. P680 then goes into a positively charged oxidized state (P680+). For the system to work, it is essential that P680+ recovers each individual electron that was given. It does this by oxidizing water, according to the following reaction (Figure 1) :
2H2O (water) → O2 (oxygen) + 4H+ (protons) + 4 e– (electrons)
Oxygen production is thus accompanied by the release of protons into the lumen: one proton for each electron released by the oxidation of H2O.
From PSII to PSI. PSII transmits electrons to plastoquinone (PQ) at the stromal face of the thylakoid membrane. When receiving two electrons, PQ absorbs two protons from the stroma, thus giving PQH2. Both PQ/PQH2 forms are mobile within the membrane and participate in the Q cycle.
PQH2 diffuses towards the cytochrome b6f complex and transmits two electrons to it (Figure 1) :
- One of them reduce P700 (from PSI) via plastocyanin ;
- The other electron is used to regenerate PQH2 (thus completing the Q cycle).
During this process, the cytochrome b6f complex pumps protons from the stroma that accumulate into the lumen through the thylakoid membrane.
Balance sheet. In total, for each electron transferred from H2O to the reducing side of PS I, three protons accumulate within the lumen :
- one proton for each electron released by water oxidation by PS II (§ 1.1.) ;
- two protons for each electron transferred by the high potential chain of the cytochrome b6f complex to PS I (§ 1.2.).
1.2. Protons and cyclic electron transfer
In some cases, ferredoxin does not transfer electrons to NADP+-oxidoreductase, but to plastoquinone via a cytochrome; hence the name cyclic transfer. The electrons will then follow the chain of transporters (PQ/PQH2, Cytochrome b6f complex, plastocyanine) which will reduce the P700 of the PSI. In the same way as in linear electron transfer, the Cytochrome b6f complex acts as a proton pump between the stroma and the lumen.
The cyclic transfer of electrons thus participates in the establishment of a proton gradient essential for the synthesis of ATP without net production of NADPH.
1.3. Proton-consuming reactions in the stroma
In the last step of the linear electron transfer, electrons are transferred to NADP+ by Ferredoxin:NADP+ oxidoreductase (or FNR) located in the stroma. This reaction consumes protons from the stroma and thus helps to establish a transmembrane gradient:
2 reduced ferredoxins + NADP+ (oxidized form of Nicotinamide adenine dinucleotide phosphate) + H+ (proton) → 2 oxidized ferredoxins + NADPH (reduced form of Nicotinamide adenine dinucleotide phosphate)
2. From proton gradient to electrochemical gradient
In 1961, Peter Mitchell [4] proposed a mechanism for coupling electron transfer to ATP synthesis. He suggested that the flow of electrons through the electron transfer chain results in the establishment of a proton gradient across the membrane. ATP synthesis is driven by an inverse flow of protons along the gradient. Mitchell’s proposal was called “chemiosmotic theory“. It won him the Nobel Prize in 1978.
This gradient between stroma and lumen can be maintained because the thylakoid membrane is generally impermeable to protons. The energy inherent in the proton gradient, called the “proton driving force” (Δp, expressed in volts), drives the synthesis of ATP by ATP synthase. It consists of two components:
- ΔpH, which is the proton concentration gradient;
- Δψ, which is the difference in charge (i.e. membrane potential) across the thylakoid membrane.
Δp = ΔΨ + Z x ΔpH
When electrons are transferred, the pH of the lumen becomes acidic (pH > 4 or 5). The light-induced transmembrane proton gradient (ΔpH) is about 3.5 pH units (see Figure 1). A pH gradient of 3.5 units across the thylakoid membrane corresponds to a proton driving force of 0.20 V.
ΔΨ and ΔpH are thermodynamically equivalent for ATP synthesis. But in illuminated chloroplasts, the membrane potential is practically zero [5], because the transfer of protons into the lumen is accompanied by a transfer of ions (Cl– in the same direction as the protons or Ca2+ or Mg2+ in the opposite direction). As a result, electrical neutrality is maintained and no membrane potential is generated: in chloroplasts, therefore, almost all of the driving proton force comes from the pH gradient. Moreover, Mitchell’s hypothesis was confirmed by André Jagendorf’s discovery that a difference in pH across the thylakoid membrane of the chloroplast leads to ATP synthesis. [6]

3. ATP synthase: a nano-machine
The determination of the molecular structure of ATP-synthase allowed us to understand how it works, which had been imagined several years earlier by Paul Boyer. [7] Chloroplast ATP synthase [8] is a large protein complex consisting of two parts (Figure 2), each of which is a type of engine:
- A hydrophobic “F0” domain embedded in the thylacoid membrane. It’s an electric motor that participates in the translocation of protons from the lumen to the stroma. The proton flux in the motor rotates a circular rotor* ;
- A globular head called “F1” soluble in the stroma. It’s a chemical engine, powered by ADP.
A peripheral rod keeps F1 anchored to the stator and prevents F1 from rotating. The two parts F0 and F1 are connected to each other by an axis which functions as a rotary shaft. The rotation of this shaft leads to sequential changes in the conformation of the catalytic sites of F1 that promote ADP and Pi binding, ATP formation and ATP release (see gif animation below) according to the following reaction :
ADP3- (adenosine diphosphate) + H+ (proton) + HPO42- (phosphate) → ATP4- (adenosine triphosphate) + H2O (water) (equation 2)
ATP synthase is therefore a unique molecular machine whose overall architecture resembles that of a water mill, driven by proton flow rather than water, as shown in the following video (by HarwardX) explaining how ATP synthase works in the inner membrane of mitochondria [9] :
Unlike ATP synthesized in the mitochondrion, which will fuel all cellular reactions, the ATP formed in the stroma will be used exclusively for the reactions that take place (synthesis of carbonaceous molecules, for example) within the chloroplast itself.
Remarkably, this research has been the subject of 3 Nobel Prizes in Chemistry, awarded to Mitchell in 1978, then to Boyer and Walker in 1997.
Notes and References
Cover image. [Source: Structure of ATP synthase, by David Goodsell (2005) Molecule of the Month, doi:10.2210/rcsb_pdb/mom_2005_12; Image licensed under CC-BY-4.0]
[1] https://planet-vie.ens.fr/thematiques/cellules-et-molecules/les-roles-de-l-atp
[2] Avenson T.J., Kanazawa A., Cruz J.A., Takizawa K., Ettinger W.E. & Kramer D.M. (2005) Integrating the proton circuit into photosynthesis: progress and challenges. Plant, Cell and Environment 28, 97-109
[3] The inner membrane of the mitochondria also contains an electron transport chain that will allow the establishment of a proton gradient. See the video “Electron transport chain” by Prof. Rob Lue (HarwardX): https://www.youtube.com/watch?v=LQmTKxI4Wn4
[4] Peter Dennis Mitchell (1920-1992) is an English chemist. He was awarded the 1978 Nobel Prize in Chemistry “for his contribution to the understanding of biological energy transfer through the formulation of chemosmotic theory”. Mitchell’s theory has revolutionized our understanding of cellular bioenergetics.
[5] This is not the case in mitochondria where the contribution of membrane potential is more important
[6] Jagendorf A.T. & Uribe E. (1966). ATP formation caused by acid-base transition of spinach chloroplasts. D.D.A. Natl. Acad. Sci. USA. 55 (1): 170-177. Bibcode:1966PNAS…55…170J. doi:10.1073/pnas.55.1.170. PMC 285771. PMID 5220864.
[7] Boyer P.D. (2002) A Research Journey with ATP Synthase J. Biol. Chem. 277:39045-39061. Paul Delos Boyer (1918-2018), an American biochemist, is co-winner, with Sir John Ernest Walker, a British chemist and molecular biologist, of the 1997 Nobel Prize in Chemistry for “elucidation of the enzymatic mechanism underlying the synthesis of adenosine triphosphate (ATP)”.
[8] This protein belongs to a large family consisting of (a) type F complexes found in mitochondria (site of respiration), chloroplasts (which perform photosynthesis) and bacteria; (b) type V (vacuolar) – found in the intracellular compartments of eukaryotes – and (c) type A (Archean), found in Archaea and some bacteria.
[9] ATP synthase in action, by Prof. Rob Lue (HarwardX)
To know more about it
- An animation of the proton transfer can be viewed on the SUN Chloroplast E-book website
- An animation of the ATP synthesis can be viewed on the SUN Chloroplast E-book website
- https://www.nature.com/scitable/topicpage/why-are-cells-powered-by-proton-gradients-14373960/
- https://www.ncbi.nlm.nih.gov/books/NBK22519/
- https://www.ncbi.nlm.nih.gov/books/NBK22352/#A2498
- David Goodsell (2005) Structure of ATP synthase par, Molecule of the Month, doi:10.2210/rcsb_pdb/mom_2005_12
- http://wwwa.unine.ch/bota/bioch/cours/ATPsynthase.html
- http://www.ipubli.inserm.fr/bitstream/handle/10608/594/MS_1997_12_1525.pdf?sequence=2sAllowed=y
- https://www.nobelprize.org/uploads/2018/06/mitchell-lecture.pdf
- https://planet-vie.ens.fr/thematiques/cellules-et-molecules/les-roles-de-l-atp