Soil reinforcement: techniques that have become essential
PDF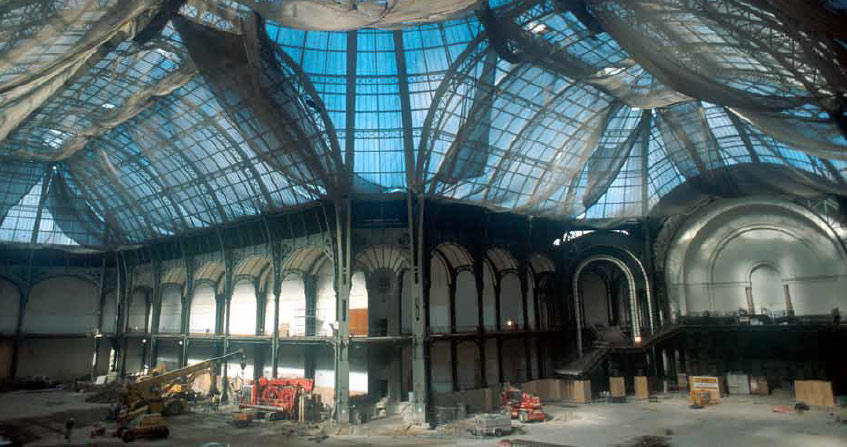
Thanks to innovative techniques, it is now possible to build on all types of floors. From simple structures to architectural feats, the know-how of engineers is mobilized to analyze, study, reinforce and consolidate soils. The geotechnician probes and determines the characteristics of the soil in order to design, within design offices, the foundations of the structures. Soil reinforcement, although generally invisible, is the cornerstone of any structure built on soil with poor mechanical characteristics. Current technological developments in soil reinforcement are moving towards more economical and environmentally friendly technical solutions.
The need for poor quality land development is increasingly necessary, given the growth of humanity and its activities. Certain types of soil, hitherto neglected because of their poor mechanical characteristics, must be reinforced to ensure the stability of the buildings and the civil engineering infrastructure they are required to support. Not very visible to the layman because they are buried in the ground, reinforcement techniques are very widespread and surround us on a daily basis (foundations, retaining walls, road embankments, railways, etc.). More than a technological challenge, the construction of infrastructure in classified unbuildable areas represents a real strategic, economic and environmental challenge in many common cases such as:
- to build a high-speed line (HSL) in an area of compressible ground, when this is more economically advantageous than diverting the line (for example, SEA HSL crossing the Dordogne),
- to install warehouses in a port area in which the soils are often highly compressible due to coastal geology (e.g. Le Havre harbor),
- to build an airport on an artificial island in a marine environment (for example, Kobe airport in Japan).
1. Techniques adapted to the complexity of the soil and each field of use
Soils are complex materials made of a more or less compact mineral skeleton with a natural porosity where air and water circulate freely. Soil grains result from the more or less coarse fragmentation of rock into pebbles, gravel, sand or silt, but also from the chemical decomposition of some rock compounds into microscopic clay particles. In permeable soils, such as pebbles, gravel and sand, water circulates easily. This is not the case in low-permeability or even impermeable soils, such as silt and clay. Water circulates 1 million times less quickly in clay than in gravel. In addition, as a result of external stresses such as water inflows, drought or earthquakes, the mechanical characteristics of soils may change and no longer be adapted to their intended use.
Depending on the type of soil considered and the type of structure to be built, it is important to choose an appropriate reinforcement solution that is adapted to both the nature of the soil in place and its environment. The problem remains highly complex, as evidenced by the remains of the past (Pisa Tower, for example). Two major techniques can be used to increase the mechanical characteristics of soils: by modifying the internal structure of the existing soil and by reinforcing the soil by adding inclusions. More specifically, soil improvement techniques can increase the compactness of the existing soil, either by reducing the volume of voids, for example by applying an overload to saturated soil and allowing it to settle by expelling the overpressure water, in this case consolidation, either by imposing vibrations in the soil so that it densifies by rearranging the grains dynamic compaction technique allowing to densify the soil to a depth of less than 10m, under the impacts of a mass of 15 to 150 tonnes falling from 20 to 40 m). Soil reinforcement techniques themselves involve vertical or horizontal reinforcement elements in the soil. The objective of all these techniques is to allow the construction of a structure without excessive surface deformation or stability defects.
2. Various and varied solutions
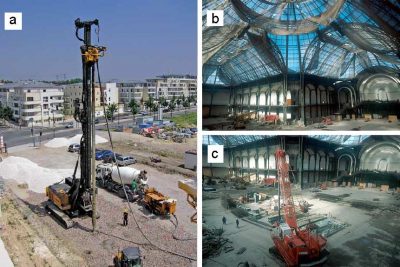
2.1. Reinforcement of the soil in place
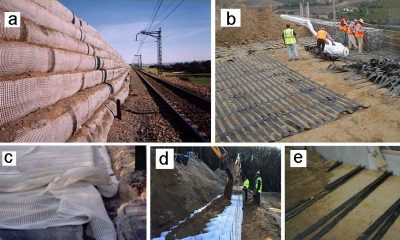
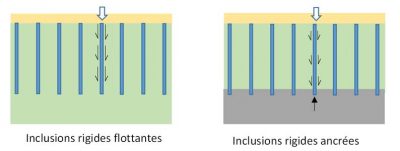
Depending on the thickness of the compressible soil area concerned, the piles can either be anchored foot in a resistant soil layer when the poor quality soil thickness is low, in which case the pile head forces are transmitted directly via the pile to the stable area (anchored piles), or of sufficient length to transmit by friction over its entire length all the loads applied at the pile head by the structure (Figure 3).
These basic techniques have evolved into many variants, all of which aim to significantly reduce soil surface settling in place, such as :
- Rigid concrete inclusions, made by inserting into the ground a driving auger (by rotation or vibrating action to introduce a tool into the ground by a simultaneous vibration and driving action) to the supporting soil layer and by gravity injecting the concrete through the foot of the tool when the tool is raised,
- Ballasted columns, undoubtedly the most flexible, made of granular materials, without cohesion, placed by displacement in the ground and compacted by successive passes,
- Jet Grouting columns, made by destructing the soil at depth using a high-pressure jet in a borehole and mixing the eroded soil with a self-hardening grout to form columns in the ground,
- Soil Mixing columns, made by mixing between the soil in place and a binder (dry track) or grout (wet track) forming a cylindrical vertical column.
Finally, the current techniques that are highly successful because they are economical or easy to implement are the so-called rigid inclusion techniques whose effectiveness is improved by the presence of a granular platform reinforced or not by reinforcement layers placed under the structure. This technique makes it possible to limit the vertical loads transmitted to the compressible ground to reasonable values, to redirect the vertical loads brought by the structure to the piles via load transfer mechanisms while minimizing the horizontal components of the load that could affect the integrity of the piles (Figure 4).
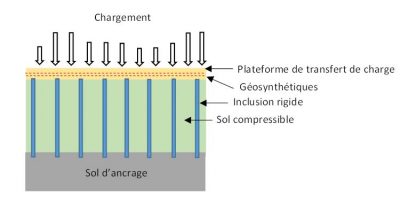
From an environmental point of view, these solutions are interesting because they do not require excavation of the soil in place, thus reducing pollution related to the transport of materials and the risk of moving polluted soil. The contribution of geosynthetic sheets [plastic, permeable (geotextiles or related products) or impermeable (geomembranes) synthetic materials used in Civil Engineering] allows to perform different functions: separation, protection, reinforcement, drainage, filtration, sealing, anti-erosion, etc. Their use in granular platforms reduces their thickness and therefore the need for “noble” materials.
2.2. Reinforcement of backfill soils
The reinforcement of backfill soils by intrusive elements mixed with the ground or arranged in alternating layers, is not new since already in -2100, in Mesopotamia, artificial constructions, ziggurats, whose realization required an enormous effort in material and manpower, were reinforced by straw weaves. The first ziggurat, built in Uruk, served as a model for all the terrace towers that were built in Mesopotamia for decades. The best preserved is that of Our (end of the 3rd millennium). Since then, there have been many developments in reinforcement techniques, particularly through the use of new types of reinforcement.

It was on a beach in 1957 that the French inventor of the Armed Land [2], Henri Vidal, made a statement: when you walk on a pile of sand, it collapses and the grains scatter in all directions, but when you insert rows of pine needles, the whole thing remains stable even if you put a lot of effort into it, for example. Henri Vidal developed this idea to develop a new technique for the construction of retaining walls, which later diversified into the form of:
- Reinforcement by flat, metallic (reinforced earth) or synthetic reinforcements placed horizontally and at regular intervals.
- Reinforcement by geosynthetic layers (Figure 5).
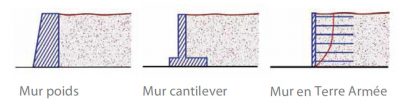
In both cases, the vertical facing does not have a supporting function but provides protection against erosion. It should be noted that the stability of the structure is ensured by the reinforcements which are opposed by traction to the deformation of the ground mass and that the tensioning of the reinforcements is achieved by friction between the reinforcement elements and the ground. This type of support is revolutionary in itself by its design since it is the reinforced ground that ensures its own stability; there is therefore no need to build a support wall that serves as a stop to the floor block – Weight wall or cantilever wall. A Cantilever wall is a reinforced concrete support wall consisting of a footing and a wall. The advantage of this type of wall compared to a conventional wall is that the floor that rests on the rear part of the footing contributes to its own stability (Figure 6).

In addition, short or continuous fibres are sometimes but more rarely used to reinforce soil masses (Figure 8). They are homogeneously mixed in the soil and act as a local reinforcement. Due to the ability of the soil/fibre mixture to dissipate energy, this technique is used, among other things, to limit the damage that could occur following the explosion of a gas tank. Here again, the tensioning of the reinforcement elements is possible thanks to the friction between the fibres and the grains or by tightening the grains in the case of long fibres.
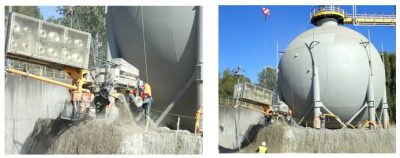
3. Complex mechanisms at work
In fact, these reinforcement techniques use complex mechanisms of interaction between soil and reinforcements that require certain simplifying assumptions to be modelled by analytical or numerical calculations. It should be remembered that the interface zone between the ground and the reinforcement is the preferred vector that allows the transmission of forces from the ground to the reinforcement, a totally smooth interface resulting in a non-transfer of forces and therefore in zero reinforcement.
What can we say about the interface? It is an area without thickness or the thickness of a few grains where the forces induced in the soil are transmitted to the reinforcement. For the maximum friction to be mobilized at the interface, it is necessary to generate a significant relative displacement between the reinforcement and the ground (at least a few millimeters or even a few centimeters depending on the materials present). The roughness of the interface influences the intensity of the forces that can be transmitted. Most often a friction angle called the Coulomb friction angle is used to characterize the interface. Alternating loading cycles (relative positive or negative displacements) modifies the intensity and direction of the forces, making it difficult to predict the effectiveness of the reinforcement during cyclic loads (wind effects on a wind turbine, repeated passages of vehicles or trains, freeze/thaw cycles, swelling/removing of the ground, etc.).
What can we say about inclusions? While their role is obvious, that of reinforcing the soil, their mode of action is sometimes complex and very different from one reinforcement to another. Geosynthetic fibres and sheets are mainly subjected to tensile forces (no compression or bending). Geogrid reinforcements with a certain bending stiffness accept both tensile and bending forces (force perpendicular to the plane of the geogrid). Vertical reinforcements of the pile type are essentially subjected to compression forces even if horizontal forces are tolerated in certain techniques.
As for the soil itself, its behaviour over time can be complex. For example, mechanisms are being developed to transfer loads from weak areas to more resistant areas. We speak of a vault effect above the pile networks.
4. State-of-the-art techniques still in development
Optimizing these techniques and reducing production costs remains a challenge that mobilizes researchers around the world. Recent techniques, new reinforcement materials, changing climatic and soil conditions are all new elements that must be constantly reconsidered. Fortunately, the means of investigation are evolving in parallel, particularly in terms of sizing. While the calculation methods for conventional structures are widely tested and validated, in complex cases engineers are increasingly using elaborate numerical models. The exponential development of computer capacities makes it possible today to make calculations that would have been impossible to implement a few years ago.
The most developed continuous methods in engineering are finite element or finite difference methods that allow to solve numerically differential and partial differential equations. The environment to be studied is subdivided into different domains (mesh made up of elements) on which the equations of the problem are solved. The choice of mesh size, type of elements and behavioural models determine the relevance of the solution obtained. Each element of the reinforcement and interface can be taken into account. Subject to calculation assumptions concerning the values of soil parameters, the results obtained are quite realistic.
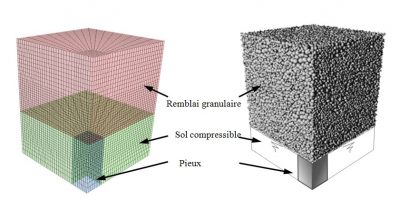
Can we dream of a digital model that takes into account each soil grain and each reinforcement element? In fact yes and some are already dreaming because discrete digital methods are more recent and come from contact dynamics or molecular dynamics. The environment to be studied is subdivided into a set of particles of various independent shapes that interact with each other at their points of contact and whose behaviour is governed by Newton’s second law. Interaction laws generally require few parameters and allow for a variety of fields of study. Unlike continuous models, these models are very well adapted to the very high deformations, cracking and fracturing of the medium under study. Each soil grain can be modelled by elements of adequate shape and interaction laws, defined between the grains and the reinforcements, make it possible to reproduce with very few parameters the behaviour of soils and reinforced structures. Very interesting to understand the local mechanisms that interact at interfaces and in the ground, these models are widely used in research. Their application to structures or parts of structures is less frequent given the technical nature of the method and the calculation times which can currently be prohibitive. Within a few decades these prohibitive computing times will be the subject of a joke.
What about the experimenters in all this? They are not to be outdone since instrumentation techniques and sensors are also evolving. Quantities that could not be measured in the past are now available for a better understanding of the reinforcement mechanisms. Model models or centrifuged in the laboratory and full-scale structures are currently being studied extensively with sophisticated instrumentation.
5. Structures under surveillance
Due to their technical nature, reinforced structures are complex structures that must be monitored over time, particularly when the economic and environmental challenges they raise are significant. In this case, the geotechnical engineer has an important arsenal of tools and measuring instruments at his disposal to analyse and anticipate the behaviour of the reinforced structure. The instrumentation of a structure can be integrated from the design stage, it makes it possible to control the behaviour of the structure during the work and to validate the dimensioning; in some cases, the instrumentation can be used to optimize the dimensioning during the construction of the structure, in this case we speak of an observational method. From the instrumentation of a structure, an SHM (Structural Health Monitoring) approach to the design and maintenance of a geotechnical structure makes it possible to anticipate structural damage to prevent accidents. Backed by statistical processing systems, they also make it possible to optimise the life of the products, while reducing maintenance costs. This approach provides, beyond the control processes alone, for the implementation of an automated and planned monitoring strategy in order to control the reinforced structure during its service life. SHM approaches are being developed thanks to the introduction on the market of new generation sensors (measurements by optical fibres in particular) and the development of means of communication and measurement transfer by autonomous acquisition centres.
6. What does the future hold for us?
What we know is that man has in his genes a desire to conquer and that he will seek to establish himself wherever he can, especially in areas hitherto reputed to be unbuildable and probably on the seabed. Increasingly concerned about its environment, it will use new, more economical and ecological techniques. In this perspective, researchers are currently developing coupling methods between numerical modelling and experimentation to better understand soil behaviour and soil-inclusion interfaces and thus lead to optimized reinforcement solutions. The observational methods already mentioned will find new outlets thanks to the development of geotechnical auscultation. Finally, man will be able to count on new allies as unexpected as bacteria, for example, which are already used to improve soil bearing capacity (biocalcification technique) or to contribute to their depollution. Very promising prospects today!
References and notes
[1] Armater : http://enka-solutions.com/en/home/
[2] Armed Land – http://www.terre-armee.fr/TA/wtaf_fr.nsf
[3] Texsol: http://www.eiffageinfrastructures.com/home/produits/texsol.html
The Encyclopedia of the Environment by the Association des Encyclopédies de l'Environnement et de l'Énergie (www.a3e.fr), contractually linked to the University of Grenoble Alpes and Grenoble INP, and sponsored by the French Academy of Sciences.
To cite this article: VILLARD Pascal, BRIANÇON Laurent (January 5, 2025), Soil reinforcement: techniques that have become essential, Encyclopedia of the Environment, Accessed April 10, 2025 [online ISSN 2555-0950] url : https://www.encyclopedie-environnement.org/en/soil/soil-reinforcement-techniques-become-essential-2/.
The articles in the Encyclopedia of the Environment are made available under the terms of the Creative Commons BY-NC-SA license, which authorizes reproduction subject to: citing the source, not making commercial use of them, sharing identical initial conditions, reproducing at each reuse or distribution the mention of this Creative Commons BY-NC-SA license.