Plants and lichens sentinels of air quality
PDF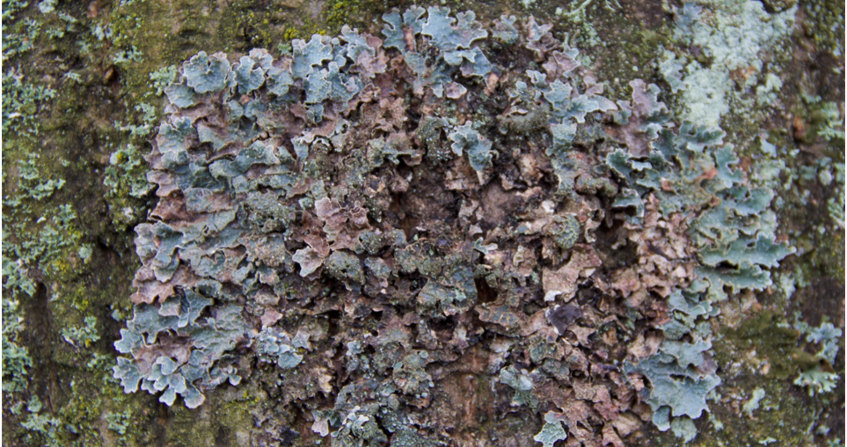
Air pollution has effects on health and the environment. The impact of pollutants on terrestrial ecosystems can be monitored and assessed by observing plants, mosses and lichens at different scales. This concept has existed since the 19th century and methods have since developed widely and have been able to adapt to the constant evolution of air pollution. Nowadays we have several approaches to observe the impacts of pollutants on plant communities, on the physiology of the different model species and even on the expression of some of their genes. Biomonitoring is a constantly evolving discipline that can adapt to current challenges such as those posed by climate change
Air pollution has significant health and environmental impacts. The health consequences are the subject of numerous epidemiological and toxicological publications that show not only effects on the cardiovascular system but also, and increasingly, on pathologies such as diabetes or neurodegenerative diseases. Environmental impacts have also been studied for a long time. Thus, travel accounts contain observations of pollution damage to forests, as well as many paintings present scenes where the sources or impacts of pollutants can also be observed. Closer to home, in the 1980s, forest dieback made it possible to raise awareness at European level of the problem of air pollution by sulphur dioxide, its environmental impacts and to contribute to raising awareness of the transboundary nature of pollution. Since then, examples have multiplied, until today with many questions about global changes. Observation of the effects of air pollution on the environment has thus made it possible to develop monitoring tools that complement physico-chemical approaches. Gathered under the term biomonitoring, many of them are based on the use of plants and lichens are true sentinels of air quality.
1. Biomonitoring of air quality: concepts and applications
Biomonitoring of air quality is therefore the use, at different scales, of living organisms such as plants or fungi to monitor the effects of pollution. It appeared in the 19th century. For example, we can cite the work of Wilhelm Nylander [1], a Finnish lichenologist, who observed in Paris, for example, a link between the decline between certain lichen species [2] and the importance of air pollution. Biomonitoring has recently been defined by AFNOR as the use of biological systems (organisms and communities of organisms) to monitor changes in the environment over time and/or space.
1.1. The different concepts of biomonitoring
Biomonitoring includes different concepts (these have also recently been redefined by AFNOR [3]) such as :
- A bioindicator is an organism, or part of an organism or community of organisms (biocenosis) that provides information on environmental impacts.
While it is theoretically possible to observe the reactions of any organism, in reality those used as bioindicators must have a number of characteristics. Thus, a bioindicator:
- must be scientifically known (its biology and ecology must be controlled: nutrition, routes of exposure to pollutants, reproduction, place in the food chain, etc.),
- be related or correlated to ecosystem functions. In this way, its reactions can be linked to a larger scale at the ecosystem level,
- integrate physical, chemical and biological properties or processes of the environment,
- be able to report, in particular, on management methods and the different types of environmental pollution,
- have measurement qualities (accuracy, reliability, robustness),
- be validated (know the amplitude of responses related to natural variations),
- be easy to use and not be a rare and/or protected species (ease of determination, sampling possibility).
- It is also possible to use bioaccumulative organisms that can provide information on environmental conditions and their modification by accumulating, on the surface and/or internally, substances present in the environment.
- Finally, an effect indicator is an organism that can provide information on environmental conditions and their changes, either through the manifestation of specific symptoms (molecular, biochemical, cellular, physiological, anatomical or morphological) or through its presence/absence in the ecosystem.
1.2. Scope of use of air quality bioindication
Air quality is continuously monitored through a system of sensor networks managed by Authorized Air Quality Associations. The fundamental tasks of these associations are to measure the concentrations of regulated pollutants, to monitor that these concentrations do not exceed the thresholds set by the regulations and to inform the authorities and the general public about air quality.
Plant and fungal biomonitoring concerns the effects of air pollutants on plants (such as tobacco, ryegrass, petunias, etc.) or corticultural lichens. It is based on the observation of the reactions of these organisms, in their environment and their responses to their exposure to pollutants. Biomonitoring provides information that is perfectly complementary to the physico-chemical measurements of sensors that provide information on the concentrations of pollutants in the air.
In many cases, organisms used in biomonitoring develop in the environment studied (passive biomonitoring). Nevertheless, when they are absent from this environment, it is possible to bring organisms (plants, mosses, lichens…) to sites by transplantation (active biomonitoring). There are many different transplant techniques, some of which are standardized. It should be noted that transplants are either cultivated organisms (this is the case with higher plants) or collected in an unpolluted environment (this is the case with mosses and lichens that cannot be cultivated).
2. Examples of applications of plant and fungal biomonitoring
2.1. The evolution of licentic and plant communities
The first “modern” observations focused on the evolution of the licene communities. In the 1970s and 1980s, the main air pollutants in Western Europe were sulphur dioxide and suspended dust (SO2 and PS), mainly of industrial origin. At that time, different authors such as Hawksworth and Rose [4] in England or Van Haluwyn and Lerond [5] in France studied the impact of these pollutants on epiphytic licene communities (developing on tree trunks, Figure 1). These authors observed a clear impact of air pollution on the specific composition of lichenic communities (decrease in specific richness but also modification of the composition of lichen populations observed on trunks with an increase in pollution), as did Nylander previously mentioned. They have developed different methods for assessing the effects of air pollutants directly in the field, through the observation of lichen species. These methods were based in particular on the observation of the presence and abundance of species more or less sensitive to pollutants. The air pollution situation has gradually evolved and become more complex (decrease in SO2, increase in concentrations of nitrogen, ozone, organic compounds, etc.).
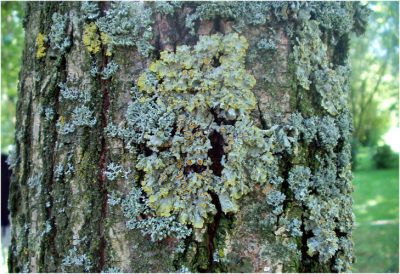
The evolution of plant, fungal and animal communities is also linked to climate change. By changing environmental parameters, this causes a change in the ranges of different species (progression at altitude, northwards, etc.) that can be monitored. This is a more recent application of biomonitoring.
2.2. Impregnation of sentinel organisms
Some organisms have the ability to accumulate pollutants in their tissues without significant physiological impacts. This property is used to assess the contamination of the environment by pollutants. This is certainly the most widely used approach to biomonitoring today. Not all pollutants can be tracked in this way, only those that can accumulate in organisms significantly are. These include metals, radioactive elements, persistent organic pollutants (dioxins, furans, PCBs, etc.), certain nitrogen compounds, etc. The quantity of pollutants accumulated reflects the bioavailable portion. Care must therefore be taken not to quantify air pollution directly from observations. These concentrations show the impregnation of the environment and also make it possible to study the transfer of pollutants within food chains and thus contribute to the assessment of health risk. This is particularly the case when contamination is assessed with plants directly consumed by humans. Methodologies, such as the BARGE protocol, make it possible to calculate the health risk on the basis of the consumption of plants contaminated by metals, to evaluate the part that will be absorbed by the body and thus to evaluate the health risk according to the toxicity of the pollutants.
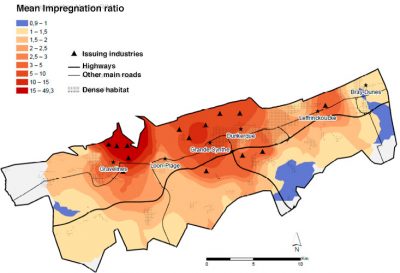
2.3. Biomonitoring based on the observation of symptoms in plants
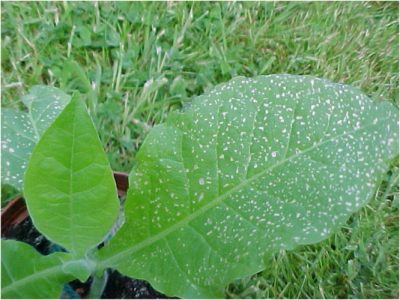
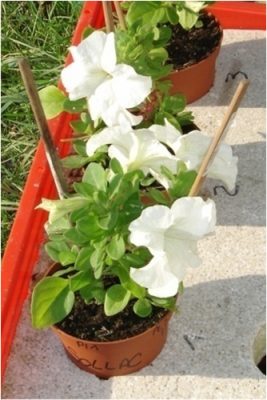
A more detailed explanation of the protocol is available on the website of APPA, the Association for the Prevention of Air Pollution.
Also to assess the effects of ozone, it is also possible to monitor the growth of clover plants (Trifolium repens L., variety “Regal” sensitive ecotype NC-S compared to the more resistant ecotype NC-R). In this case, ozone causes a slowdown in growth. However, ozone is not the only pollutant whose effects may be visible. Thus, Petunia hybrid can be used to highlight the effects of volatile organic compounds (Figure 4). These pollutants modify the growth and flowering parameters of exposed plants.
2.4. Going from the visible to the invisible
Nowadays, the effects of pollutants are most often invisible to the naked eye. Moreover, when visible damage occurs, it is often “too late”. For many years, work has been carried out to develop biomarkers of plant exposure and/or effect to pollutants. These biomarkers are of very diverse natures, combining the monitoring of physiological responses (cell respiration, photosynthesis, etc.) with the monitoring of compensation mechanisms (enzymatic or non-enzymatic antioxidant systems, etc.) or effects (DNA breaks, formation of micronuclei, “comets” as shown in Figure 5, etc.).

The objective of all the methods mentioned in this paragraph is to develop early indicators of the effects of pollutants but also specific to pollutants.
3. Points to remember
- Plants, mosses and lichens are used as indicators of the impacts of air pollution on the environment.
- It was the lichens growing on tree trunks that paved the way for biomonitoring. The methodology used today determines the Biological Lichens Epiphyte Index (IBLE) which is based on the diversity, frequency and coverage of lichens on trunks. This index is related to the impacts of air quality.
- Some organisms have the ability to accumulate pollutants in their tissues without physiological impacts. They make it possible to carry out spatio-temporal monitoring of the impregnation of the environment by these pollutants.
- Ozone has the particularity of causing necrosis on plant leaves. They are particularly observable on tobacco leaves, other pollutants affect the growth of clover or the flowering of petunias. These model plants are used in biomonitoring approaches.
References and notes
Cover image. Parmelia sulcata: foliated lichen commonly used in biomonitoring for its ability to accumulate trace elements. [Source : © Damien Cuny]
[1] Nylander, W. (1896). Lichens from around Paris. Librairie des Sciences Naturelle, Paul Klincksieck, Paris.
[2] Lichens are organisms formed by the symbiosis of a fungus and an algae. They belong to the kingdom of mushrooms. Without roots, stems, leaves, their vegetative organism is called a thall. They depend on exchanges with the atmosphere for their water and mineral nutrition. Photosynthesis carried out by algal cells within the thallus allows the synthesis of organic compounds.
[3] Leblond S., Gombert-Courvoisier S., Louis-Rose S. (2014). Standardization in the field of air quality biomonitoring. International Workshop on Plant and Fungal Biomonitoring of Air Quality, Lille, France, 13 & 14 October 2014.
[4] Hawksworth, D.L. Rose, F. (1970). Qualitative scale for estimating sulphur dioxide air pollution in England and Wales using epiphytic lichens, Nature, 227, 145-148.
[5] Van Haluwyn C., Lerond M. (1986). Lichens and air quality: methodological evolution and limitations. Report n°2130, Paris, Ministry of the Environment (SREIE).
[6] AFNOR (2008). Air biomonitoring – Determination of the Biological Index of Lichens Epiphytes (IBLE), Standard NF X43-903. Paris, AFNOR.
[7] AFNOR (2008). Biomonitoring of the air – Bioindication of ozone by tobacco, Standard NF X43-900. Paris, AFNOR.
The Encyclopedia of the Environment by the Association des Encyclopédies de l'Environnement et de l'Énergie (www.a3e.fr), contractually linked to the University of Grenoble Alpes and Grenoble INP, and sponsored by the French Academy of Sciences.
To cite this article: CUNY Damien (January 5, 2025), Plants and lichens sentinels of air quality, Encyclopedia of the Environment, Accessed April 12, 2025 [online ISSN 2555-0950] url : https://www.encyclopedie-environnement.org/en/air-en/plants-lichens-sentinels-air-quality-2/.
The articles in the Encyclopedia of the Environment are made available under the terms of the Creative Commons BY-NC-SA license, which authorizes reproduction subject to: citing the source, not making commercial use of them, sharing identical initial conditions, reproducing at each reuse or distribution the mention of this Creative Commons BY-NC-SA license.