Dialogue and cooperation among bacteria
PDF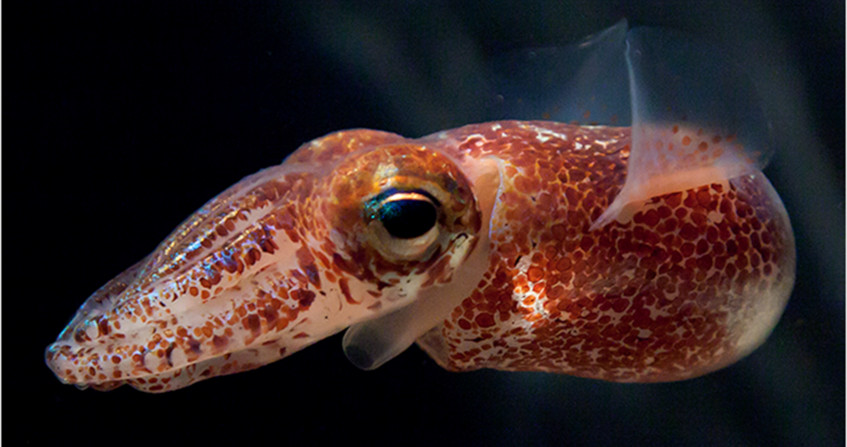
Are social communication and cooperation the prerogative of animals? Absolutely not: they also exist in plants and unicellular organisms, such as bacteria. Among the latter, social behaviour depends in particular on the local density of individuals. Thus the bacteria Vibrio fischeri, among other species, have a density-dependent communication system, called “quorum sensing”, which allows them to coordinate their activities and cooperate at high cell density. How does this system work? Why and how did it evolve? Is it widespread in bacteria, and does it exists in other microorganisms? What are the responses of host species to quorum sensing of pathogenic bacteria? Can the understanding of quorum sensing mechanisms help microbiologists control microbial attacks, such as biofilms formation by pathogenic bacteria? These are some of the questions explored by researchers in evolutionary biology and microbiology since the turn of the century.
- 1. Host – microbial associations: a complex ecosystem
- 2. A historical model: the bioluminescent bacteria of cuttlefish Euprymna scolopes
- 3. The multiple uses of quorum sensing
- 4. Evolution and adaptation of quorum sensing
- 5. Diversion and exploitation of the signal
- 6. Manipulating molecular dialogues
- 7. To collaborate, or not? That is the question…
- 8. A system to explore, in multiple areas!
- 9. Messages to remember
1. Host – microbial associations: a complex ecosystem
Be they plants, animals, algae or fungi, all multicellular organisms (or macroscopic organisms that we distinguish with the naked eye) host on their surface and in certain organs abundant microbial communities called “microbiotas“, including bacteria, protists* (such as amoebas and unicellular fungi), and possibly archaeae*. Such associations between macro- and micro-organisms are of course not neutral for the parties –together between them and with the host species for many generations, some or all of the species that are members of a microbiota can contribute significantly to the physiological functioning of their host (also known as “macrobiont”), thus establishing a relationship of mutual dependence or symbiosis* with it (See Symbiosis and parasitism). This is the case of the human gut microbiota, recently popularized by Giulia Enders’ book “Gut“ [1] and described in another article in this encyclopedia (see Human microbiotas: allies for our health).
Host and microbiota are thus allied… to a certain extent! If the relationship is not always beneficial to both parties, it is mainly because, unlike the first one -issued from the division of a single cell-, the second one is not an individual organism, nor a “super organism” acting in tune with each other. Belonging to many different species, the microbiota cells are involved in multiple ecological and metabolic relationships, both between themselves and with their host. They synthesize or degrade and secrete in the external environment many chemical compounds, which have various effects on other surrounding cells, as well as on their host. They can thus communicate with each other via chemical signals (known as pheromones) and cooperate by pooling molecular tools (such as “digestive” enzymes); but also fight each other via the emission of toxic compounds for example [2]. All activities that usually stabilize the mutualist relationship* between the host and his microbiota, but sometimes also lead to a regulated attack on the host by the latter!
The “molecular dialogues” between cells of a microbiota are complex, as shown by the researches of the last twenty years in this field. Among the chemical signals/messages emitted by bacteria, some stimulate the cooperation of receiving bacteria only under certain conditions (of density), according to a mechanism called ‘quorum sensing‘. From a microbiological point of view, applied to medicine and public health, understanding the mechanisms involved in this conditional cooperation – which often results in an increase in the virulence and toxicity of the bacterial populations concerned, see below – opens up many opportunities. More broadly, on a theoretical and evolutionary level, the study of the mechanisms and issues of bacterial quorum sensing allows to explore the evolution of cooperation in bacteria [3].
2. A historical model: the bioluminescent bacteria of cuttlefish Euprymna scolopes
When it hunts in moonlight, the bioluminescence of the photophores adjusted to the intensity of the apical lighting allows it to compensate for the lack of light on its ventral surface and to mask its cast shadow. Thus, paradoxically, emitting light allows the sepiola to hide its presence from its enemies, predators and prey [4]. A very adaptive behaviour! But the light-producing cells in the photophores do not belong to cuttlefish: they are symbiotic bacteria of the species Vibrio fischeri, capable of bioluminescence [5], which coordinate their light production under the control of their host’s neuroendocrine system… after having contributed to its embryonic development [4]! Behaving so, these bacteria increase the probability of survival of their host, its reproductive success, and hence their own selective value*!
In the early 1970s, it was shown that Vibrio fischeri bacteria only synthesize the luciferase enzyme needed to produce light when their concentration reaches a threshold, or quorum, that the bacteria are able to detect [5]. This phenomenon was then called quorum sensing. It refers to a (chemical) communication system between cells of a population of bacteria, or other microorganisms, by which they inform each other of their local density, and which allows the initiation and coordination of collective activities above a certain density threshold (see Focus Quorum sensing and chemical messengers). Bacteria then exhibit cooperative or ‘social’ behaviour, and are able to synchronize the expression of specific functions at the scale of a microbial cell population. In the case of symbiotic bacteria in cuttlefish, the function thus controlled is the production of light (bioluminescence).
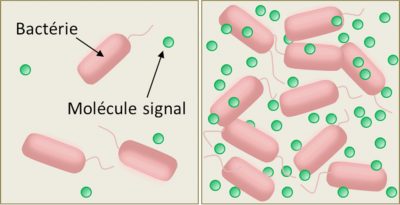
The mechanism is quite simple. Bacteria capable of quorum sensing synthesize and release into the external environment small organic molecules that act as chemical signals (pheromones), to which they are themselves sensitive (Figure 2). The binding of the chemical signal to a cell-specific receptor (membrane or cytoplasmic receptor, depending on the species) stimulates the synthesis and secretion of the pheromone – which increases the local concentration of this signal molecule in the extracellular medium – according to a process known as self-induction or self-training. That’s why microbiologists call the chemical signals of quorum sensing “self-inductive”. The local concentration of the chemical signals of the quorum sensing therefore varies with the density of the bacteria (Figure 2).
At low cell density, this concentration is very low, few signal molecules are detected before they are degraded or locally eliminated by diffusion; the functioning mode (or phenotype) of the cells therefore remains stable, of the “individualistic” type. But from a certain density threshold, due to the self-induction loop, the local concentration of these molecules causes the cells to switch to another mode of functioning (or phenotype), involving the synthesis of molecules useful to the whole group and promoting their coordination: a so-called “social” or “cooperative” phenotype.
3. The multiple uses of quorum sensing
Since the 1980s, the use of quorum sensing for synchronization and coordination of collective activities has been verified in a large number of bacteria (see for example [2],[3]). It has also been shown in archaea and in some saccharomycetes fungi with two alternative growth modes (mycelium or budding) [6],[7].
Quorum sensing controls many functions in these microorganisms, with important consequences for the functioning of the microbiota and the physiology of the host [2],[6]. For example, various research teams are investigating the role of quorum sensing in certain strains of Vibrio belonging to the microbiota of oysters and which may become pathogenic under certain conditions.
3.1. Virulence
Many microorganisms control their pathogenicity by quorum sensing: this is the case of pathogenic bacteria parasiting plants (Erwinia sp.) or mammal skin (e.g. Staphylococcus aureus), of the cholera agent (Vibrio cholerae), and probably also of the fungus Candida albicans. In other bacteria, such as Escherichia coli and Klensiella sp., quorum sensing controls the collective resistance to antibiotics provided by the production and secretion of enzymes (especially lactonase*) capable of breaking antimicrobial molecules.
3.2. biofilm formation
4. Evolution and adaptation of quorum sensing
How can we explain the existence and evolution of such a social communication system in many bacteria, archaea and other microorganisms living in isolated habitats such as microbiomes? In an ecological, genetic and evolutionary approach, it can be stressed that the formation of dense microbial communities in local habitats rich in nutrients, combined with the usual functioning of microorganisms (feeding, reproduction, defence, communication modes…) promote the evolution of such systems.
The density. In general, cell density in microbiotes increases the frequency and diversity of chemical and biophysical interactions between species and within populations of the same species, potentially beneficial or harmful to individuals (in terms of selective value*). It therefore fosters the evolution of multiple communication systems based on these interactions, systems dedicated to cooperation or, on the contrary, to the avoidance of ecological partners [8],[9].
Isolation. Moreover, whether confined to an organ of their host or concentrated around a source of nutrients, the (even relative) isolation of microbial communities increases the inbreeding of local populations of the same “species” – i.e. in the case of bacteria, populations resulting from the cloning of individuals. It therefore promotes the evolution of social behaviour within these local populations [10],[11].
At a higher level of integration, the cells of a microbiota are engineers of their ecosystem:
- their local density is such that, through their multiple metabolic activities, they significantly modify their biophysical-chemical environment (at the scale of their host).
- in turn, environmental changes caused by the collective activity of very many cells promote the emergence, evolution and control of adaptive social behaviours for individuals in high-density conditions [2],[3].
Like all species-specific behaviours, or certain categories of individuals within species – juveniles, for example, or breeding adults in animals – communication signals and social behaviours do evolve only if they increase the selective inclusive value* of the individuals who perform them. This is the case for the collective hunting of wolves or the collective defence of meerkats, for example, which in both cases involve only adults and subadults (mostly related to the juveniles in the group). The same is true for bacterial activities controlled by quorum sensing… on another numerical scale!
To be effective at the scale of their sepiola host (or at the scale of the sepiola-bacteria holobionte), light emission by V. fischeri bacteria must be massive and synchronized. Luciferase synthesis and reaction chains involved in light emission, however, are physiological activities that are very costly in terms of energy, penalizing (adaptively) when they have no effect, as in the case of few bacteria or/and poorly coordinated. In short, these activities are only adaptive for bacteria under certain density conditions.
Similarly, the synthesis and secretion in the external environment, by bacteria in “social” mode, of large energy-intensive molecules such as enzymes, polysaccharides, siderophors and other “molecular tools” useful to the community, only benefit secretory bacteria (in terms of inclusive selective value*) if they are surrounded by many cells acting in the same way, often from the same clone. In other words, it is about producing and making available to other cells “public goods” that are costly to produce (cf. [2]), activities that can only be counter-selected in the absence of reciprocity.
Under these conditions, a system of reciprocal communication and stimulation allowing potential collaborators to learn about their local density – via the emission and detection of small signal molecules – and to cooperate at high density can only be promoted by natural selection, since it confers a selective advantage to the performers. In short, quorum sensing allows bacteria to cooperate if and only if biological (genetic, ecological) conditions are favourable to the participants… unless the system is diverted!
5. Diversion and exploitation of the signal
In microorganisms, as well as in animals or plants, any new communication system can emerge and evolve only if it promotes functional interaction between the parties (reproductive partners, social congeners, ecological partners, food or sexual competitors, etc.), to the benefit of each of them (see for example [9],[10]). The communication partners can then be designated as the sender and the “biological recipient(s)” of the signals.
Biological emitters and ‘receivers’, however, do not live alone in their habitats: they are in contact with many other organisms, potential detectors and users of any information (and any potential material resources) conveyed by the chemical, visual, sound and other manifestations of the species present. The song of a male nightingale perched on a branch, for example, is heard as much by the hunting marten and human walkers as by the members of its species to whom it is addressed (current or potential sexual partner, sexual rivals, etc.)
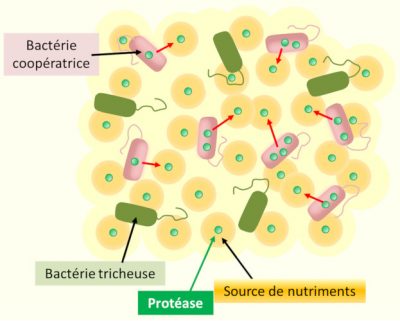
The emission of a signal only evolves if its effect on all the organisms that perceive and react to it – whether they are functional partners or not – is globally beneficial to the emitters (in terms of selective inclusive value)… in the usual living conditions of the species. This does not preclude the detection, exploitation and/or possible diversion of signals by other organisms, sharing the same habitat, for which they are not intended. And this of course applies to bacterial quorum sensing.
The existence of mutant bacterial strains, insensitive to quorum sensing signals from their congeners, has been demonstrated in several species. Dispensed with the energy-intensive syntheses of their “social” congeners, but benefiting from the enzymatic activities and molecular tools (known as “public goods”) released in the external environment by the latter, these unconditionally individualistic mutants are in the short term more competitive than their social congeners, and initially spread into populations. In other words, they are “cheaters”, who parasitize a system of communication and cooperation to which they do not contribute (Figure 4). [12]
The increasing fraction of these “cheaters”, however, gradually reduces the local density of social mode cells. It therefore reduces both the productivity of the group (hindering the collective functioning of the local population) and the density of quorum sensing signals, which ultimately puts an end to local cooperation [13].
The exploitation of quorum sensing signals emitted by bacteria is of course not the exclusive domain of “cheating” mutants insensitive to these signals, appearing from time to time in bacterial lines. In theory, natural selection should favour the use -or misuse- of a signal in any species frequenting the same habitat likely to perceive that signal, and in the host species itself, as long as this use increases the average reproductive success (or, more precisely, the inclusive fitness*) of the users. This allows the exploitation of a pre-existing communication system by out siders, the evolution of which will in turn exert a selection pressure on the biological emitters and/or recipients of the signal (see e.g. [9],[10] and see below).
6. Manipulating molecular dialogues
The compounds involved in quorum sensing control important functions in microorganisms that are often harmful to humans, such as pathogenicity or the ability to form biofilms. Many research teams aim to manipulate these molecular dialogues to control, limit or stop these microbial activities. The challenge is all the more important as the resistance of microorganisms to known antibiotics increases. The search for alternative control strategies is therefore essential.
To achieve this objective, researchers are inspired (in a biomimetic and/or evolutionary approach) by strategies for exploiting signals observed in nature. It is presently known that many species, particularly hosts, are capable of inhibiting molecular dialogues of quorum sensing between microbial cells: this phenomenon is called quorum quenching.
The emission of a signal only evolves if its effect on all the organisms that perceive and react to it – whether or not they are functional partners – is generally beneficial to the emitters (in terms of selective inclusive value)… in the usual living conditions of the species. This does not preclude the detection, exploitation and/or possible diversion of signals by other organisms, sharing the same habitat, for which they are not intended. And this is of course the case for bacterial quorum sensing.
The applications of quenching quorum are therefore multiple, and many are still only at the experimental stage. Some teams have used Bacillus bacteria to control fish pathogens in aquaculture [15]. These bacteria secrete enzymes that degrade quorum sensing messengers. Added in the form of small balls in fish feed, they allow to control pathogens in aquaculture tanks or aquariums by degrading the chemical messengers controlling their coordination. Others are trying similar strategies to control biofilms that develop on filtration membranes in industry [16].
Some prospects seem particularly promising in medicine. It is known that antibiotics significantly modify our intestinal flora. Induced imbalances can lead to the appearance of certain pathogens and diseases. But a recent study [17] showed that injecting bacteria overproducing a quorum sensing molecule (AI-2) into a mouse’s intestine helps to control the microbial populations that develop there. Thus, after an antibiotic treatment harmful to the microflora, the injection of this strain allows to find a more diversified microbiota, in particular enriched with Firmicutes.
7. To collaborate, or not? That is the question…
Strength comes from unity, they say. This maxim seems obvious, almost a truism, in our human eyes: social behaviour, cooperation and organisation between human partners or allies allow carrying out several actions that are beneficial to all participants, but beyond the reach of independent individuals, each acting for himself.
However, even in the animal kingdom that stands out in this field, cooperation is not a golden rule. Unlike swans and most birds, Mallard ducks raise their young alone. Close cousins of lions, tigers hunt alone. If the honeybee is very social (eusocial), many species of bees are solitary… It is necessary to recognize and question the multiple roles of the physical, biological and social environment in the evolution of “altruistic” behaviour and communication [18]. The same applies to microorganisms.
Cooperation always involves costs and risks, among which those associated with the emission of communication signals and the detection of these signals by non-receiving organisms, unwilling to collaborate: competitors, predators, parasites (e.g. [9],[10]). Therefore, social behaviour and cooperation can only evolve if the benefits – in terms of selective value, i.e. the transmission of genes to future generations – exceed these costs. Hence the evolution of quorum sensing in many bacteria, which can pass from harmless to pathogenic for their host according to the effectiveness of its defences and/or the microbiotic context for example; hence the episodic but short-term expansion of “cheaters” insensitive to social signals, in bacterial populations controlling their cooperation by quorum sensing; and hence the evolution of “quorum quenching” in host or competing species of these bacteria.
8. A system to explore, in multiple areas!
The study of microbial interactions is an evolving research subject. The characterization of the chemical messengers involved and the identification of the microbial functions they govern remain challenges for current research. Beyond medical research, evolutionary biology and ecological research on quorum sensing suggest that this system of conditional cooperation plays an important role in the evolution of symbioses and other interactions between ‘microbions’ and their hosts, as well as in the adaptation of microbial communities to current environmental changes. Its study thus has its rightful place in environmental sciences, aiming to an ecological transition.
9. Messages to remember
- A key mechanism for the social behaviour of bacteria, quorum sensing is based on the emission and detection by cells of small signal molecules, or “key molecules”, whose concentration in the external environment increases with that of bacteria.
- Above a certain concentration threshold in the external environment, these key molecules cause the cells to switch to another functioning mode, of a cooperating type.
- Characteristics of each “species” or bacterial strain, the molecular signals of quorum sensing are very diverse.
- This mechanism is not specifically bacterial: it has recently been demonstrated in other microorganisms, protists and archaea.
- On the theoretical and evolutionary level, the study of quorum sensing allows to explore the evolution of social behaviour in bacteria and other microorganisms, as well as the evolution of interactions -symbioses in particular- between them and their hosts.
- Quorum sensing has been extensively studied in strains of medical interest, where it is beginning to be manipulated to limit bacterial activities harmful to humans.
Notes and references
Cover image. The small cuttlefish Euprymna scolopes (Source: Chris Frazee & Margaret McFall-Ngai [CC BY 4.0]).
[1] Enders G. (2015). Gut: The Inside Story of Our Body’s Most Underrated Organ. Greystone Books; translated from the original book in german by Enders G. (2014) Darm mit Charme : Alles über ein unterschätztes Organ, Ullstein, Berlin.
[2] West S.A., Diggle S.P. et al., 2007. The social live of microbes. Annu. Rev. Ecol. Evol. Syst. 38:53–77.
[3] Diggle S.B. et al., 2007. Evolutionary theory of bacterial quorum sensing – When is a signal not a signal ? Phil. Trans. R. Soc. B 362:1241-1249.
[4] McFall-Ngai M., 2014. Dividing the essence of Symbiosis: Insights from the squid-Vibrio Model. PLOS Biology 12(2): e1001783, doi:10.1371/journal.pbio.1001783.
[5] Nealson K., Platt T. & Hastings J.W., 1970. The cellular control of the synthesis and activity of the bacterial luminescent system. Journal of Bacteriology 104 (1):313–22.
[6] Albuquerque P. & Casadevall A., 2012. Quorum sensing in fungi – a review. Medical Mycology 50:337–345.
[7] Zhang F., 2012. Acyl homoserine lactone-based quorum sensing in a methanogenic archaeon. ISME Journal 6:1336–1344.
[8] Teyssèdre A., 1993. La Communication animale, sur la scène de l’évolution. Paris, Nathan ; Teyssèdre A., 2006. Les clefs de la communication animale. Paris, Delachaux et Niestlé.
[9] Maynard Smith J. & Harper D., 2003. Animal signals. New York, Oxford University Press.
[10] Hamilton, W. D. 1964. Genetical evolution of social behaviour I. J. Theor. Biol. 7:1–16.
[11] Maynard Smith J., 1989. Evolutionary Genetics. New York, Oxford University Press.
[12] Diggle S.P., Griffin A.S. et al., 2007. Cooperation and conflict in quorum sensing bacterial populations. Nature 450:411-414.
[13] Popat R., Crusz S.A. et al., 2012. Quorum-sensing and cheating in bacterial biofilms. Proc. R. Soc. B 279:4765–4771.
[14] Rasmussen T.B., Manefield M., Andersen J.B., Eberl L., Anthoni U., Christophersen C. et al., 2000. How Delisea pulchra furanones affect quorum sensing and swarming motility in Serratia liquefaciens MG1. Microbiology 146(12):3237-3244
[15] Defoirdt T., Sorgeloos P. & Bossier P., 2011. Alternatives to antibiotics for the control of bacterial disease in aquaculture. Current opinion in microbiology 14(3): 251-258.
[16] Oh H.S., Yeon K.M., Yang C.S., Kim S.R., Lee C.H., Park S.Y. et al., 2012. Control of membrane biofouling in MBR for wastewater treatment by quorum quenching bacteria encapsulated in microporous membrane. Environmental Science & Technology 46(9): 4877-4884.
[17] Thompson J.A., Oliveira R.A., Djukovic A., Ubeda C. & Xavier K.B., 2015. Manipulation of the quorum sensing signal AI-2 affects the antibiotic-treated gut microbiota. Cell reports 10(11):1861-1871.
[18] Krebbs J.R. & Davies N.N. (Eds), 1991, 1997. Behavioural Ecology. Oxford, Black Well Scientific Publications.
The Encyclopedia of the Environment by the Association des Encyclopédies de l'Environnement et de l'Énergie (www.a3e.fr), contractually linked to the University of Grenoble Alpes and Grenoble INP, and sponsored by the French Academy of Sciences.
To cite this article: LAMI Raphaël, TEYSSEDRE Anne (December 3, 2019), Dialogue and cooperation among bacteria, Encyclopedia of the Environment, Accessed December 27, 2024 [online ISSN 2555-0950] url : https://www.encyclopedie-environnement.org/en/life/dialogue-cooperation-bacteria/.
The articles in the Encyclopedia of the Environment are made available under the terms of the Creative Commons BY-NC-SA license, which authorizes reproduction subject to: citing the source, not making commercial use of them, sharing identical initial conditions, reproducing at each reuse or distribution the mention of this Creative Commons BY-NC-SA license.