核能利用
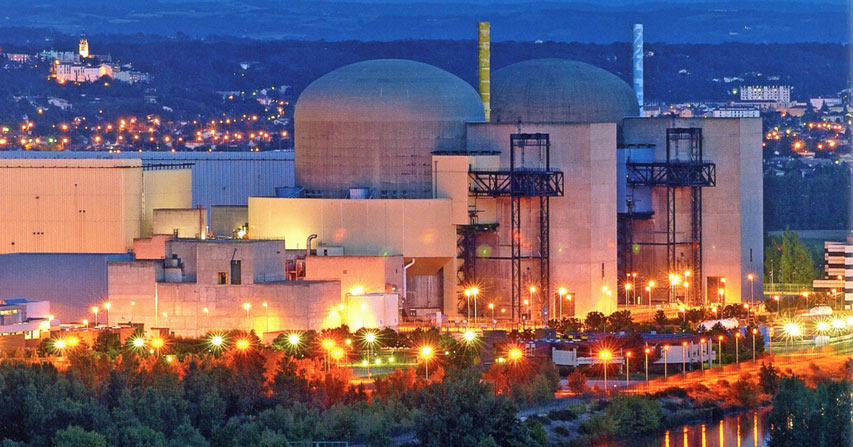
核反应堆将铀核裂变产生的热量转化为电能,其发电量可以根据需要进行调节。核反应堆正常运行时对环境非常友好,特别是温室气体排放量很低。20世纪50年代以来,核反应堆已演进了数代,每一代都从少有的几例事故中吸取教训,提高了安全性和稳定性。放射性废料的产量和体积有限,可以在很长时间内得到有效控制,但人们对废料的管理仍有争议。
全世界约有450个核反应堆利用裂变生产了世界11%的电力。在这篇文章中,我们将描述裂变反应和裂变反应堆的运行原理,以及它们对环境的正负面影响。阅读这篇文章之前,可以先读一读《放射性与核反应》这篇文章。
1. 核裂变
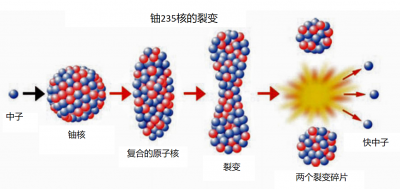
少数几种非常重的原子核(铀:U-233和U-235; 钚:Pu-239和Pu-241)在吸收一个中子后,会变得不稳定,它们通常会分裂成两部分,这就是核裂变。这些容易发生裂变的原子核被称为易裂变核。在裂变过程中,会有几个中子(通常是2或3个)高速射出(约20000公里/秒)。裂变碎片的质量总和小于初始原子核及其所吸收中子的质量总和:正如爱因斯坦告诉我们的,这种微小的质量损失对应着大量的能量释放。著名的公式E = mc2 更准确的写法是ΔE =-c2Δm,其中ΔE代表释放的能量,Δm代表最终质量和初始质量之差,c代表真空中的光速。
这种能量主要体现为分离后碎片中的动能,但是当这些碎片与相邻的原子碰撞使其产生震动时,一切动能都会转化为热量(见《什么是能量》。之后我们会介绍被称为动力堆的核反应堆是怎样利用这种热量发电的。一个基本的化学反应通常只会释放几电子伏特(eV)的能量,但一次裂变却能够释放出2亿电子伏特(200 MeV)。因此,一克铀的裂变产生的能量,比一吨石油燃烧产生的能量还要多。
一个原子核裂变后,如果裂变新产生的中子遇到其它易裂变的原子核,这些原子核可能也会裂变,引起链式反应。当这种反应呈指数型增长,会非常迅速地释放出巨大的能量,这就是原子弹的原理。但如果中子的数量保持不变,则释放的能量是稳定的,而这正是核电站要达到的效果。
1934年,恩利克·费米(Enrico Fermi)首先观察到,如果中子在入射到靶物质之前,与轻核(例如石蜡)发生弹性散射而减速,那么靶物质将吸收更多中子。这种减速被称为慢化或热化,因为中子速度降低到了慢化材料的原子核进行热运动的水平。这种现象在大多数核反应堆的运行中起着关键作用,这些核反应堆被称为热反应堆,与 “快中子反应堆”相对。
有一些重原子核,虽然本身不易裂变,但是在吸收中子后,会变为具有β放射性的新原子核[1]。这种新原子核发生β衰变后产生的“子核”却是易裂变的。这种吸收中子后可转变为易裂变核的原子核被称为“可增殖核”,如钍Th-232可增殖为铀U-233,铀U-238可增殖为钚Pu-239。
再来看裂变碎片。大多裂变碎片是放射性的,其中多数衰变得很快,变为新原子核,这些新原子核通常也具有放射性。残存未衰变的裂变碎片和这些碎片的衰变子体构成了裂变产物。绝大多数裂变产物没有利用价值,构成了所谓“高放射性废料”中的绝大部分(下文会讨论废料的管理)。除了裂变产物,放射性废料中还包括一些被核燃料原子核非裂变中子吸收的放射性重核:镎、镅和锔。它们被称为次锕系元素(MA),其长放射性半衰期[1]将引出一个问题,我们稍后会讨论。
反应堆通过链式裂变反应产生能量时,裂变产物会在堆芯积累。当链式裂变反应停止时,这些裂变产物将继续衰变,释放出相当高的剩余功率,功率起初很大,但会随着时间推移而衰减。一个典型的反应堆在停堆10秒后,剩余功率约为其标称功率的5%,一天之后为0.5%,一周后为0.3%。对于标称产生1000 MW电力的反应堆而言,0.3%的剩余功率,相当于反应堆停止运行后,仍将产生9 MW热功率(产生的热功率是剩余功率的3倍 )。这种现象是核反应堆特有的,且无疑是核反应堆的首要安全问题:反应堆停堆后,堆芯仍必须冷却数周以避免熔化,这正是下文介绍的美国三哩岛和日本福岛核事故的情况。
2. 核反应堆
2.1. 发电厂,反应堆
通常情况下,一个发电厂或电力生产中心会运行数个电力生产单元。但这里我们仍将使用“发电厂”来指代一个电力生产单元。最常见的发电厂为水力发电厂和传统的热力发电厂。热力发电厂的能量来自煤、天然气以及目前已经很少使用的重燃油的燃烧。
除了使用燃气轮机的发电厂之外,热力发电厂都是在锅炉中将液态水转化为高压蒸汽。高压蒸汽在涡轮机中膨胀,驱动涡轮机叶片转动,然后在冷凝器中回到液态。冷凝器外部通过冷却水冷却。冷凝水随后返回锅炉进行新的水-蒸汽循环。涡轮机的轴(通常由几个部件组成)固定在交流发电机的轴上,带动交流发电机旋转产生电流。所有涡轮机体和交流发电机都被称为涡轮交流发电机单元。产生的电力被输送到变电站,即增加电压的变压器,然后输送到高压输电网(电压越高,输电线路上的损耗越低)。
核电厂也是一种热力发电厂,其供给水–蒸汽循环的能量来自核反应堆核心中的链式裂变反应。
2.2. 核燃料,堆芯
与其他热力发电厂类似,虽然燃烧不是直接用于发电,但核燃料是释放发电所需热量的裂变材料。因此,核燃料必须含有易裂变的铀或钚。最常用的燃料是由氧化物粉末烧结而成的圆柱形陶瓷颗粒。
核燃料装在燃料组件中。燃料组件具有两重功能:一方面它可以将裂变产生的各种放射性元素包裹在组件内部,另一方面,它们将核裂变反应释放的热量有效地传递给传热流体(气体或液体)。这种热传递为发电设备提供热量,并反过来将核燃料组件保持在所需的安全温度。

(图中:控制集群—控制棒;上层线圈—上定位格架;导管—导向管;混合格栅—组装格栅;组件—燃料棒;下层线圈—下定位格架)
核燃料组件是根据给定的反应堆设计模型确定的,因此会有各种不同形状和尺寸的组件。一个典型的组件(见图2)由一束密封的金属管组成,燃料芯块堆叠在金属管中。这些管子被称为“燃料棒”,它们的金属外壳称为“包壳”。整个反应堆燃料包含在多个核燃料组件中,构成了反应堆堆芯。依据不同反应堆的类型,当然还有功率要求,堆芯组件的数量差异很大。
2.3. 受控的链式裂变反应
为了维持核反应堆中的链式裂变反应,在堆芯中裂变反应产生的中子数必须始终与被堆芯吸收及从堆芯中逸出的中子数之和完全相等。产生和消失的中子数之比被称为倍增系数,记为K。K必须严格等于1,这是临界条件,K=1时反应堆处于临界状态。
如果K小于1,堆芯中的中子迅速衰减消失,链式裂变反应停止,反应堆也停止运行:堆芯处于次临界状态。如果K大于1,堆芯中子的数量会快速增加(由此导致裂变反应及堆芯释放的能量也快速增加),链式裂变反应会“反应过度”,即堆芯处于超临界状态。
为了使反应堆永久保持在临界状态(K = 1),需要引入(或去除)由吸收中子的原子核组成的中子吸收剂。我们通常使用3种类型的中子吸收方法:
- 可移动(可自由插入堆芯及从堆芯拔出)的中子吸收棒,称为控制棒;
- 溶解在冷却剂中的中子吸收材料,其浓度可随时调节。这种中子吸收剂被称为均质中子吸收剂。
- 弥散在核燃料中的随反应堆运行而逐渐消耗的中子吸收材料,即可燃中子吸收剂。
2.4. 缓发中子的关键作用
链式裂变反应中两次连续裂变之间的时间间隔过短(因为即使是堆芯中最慢的中子,也在以2千米/秒的速度运动!):在如此短的时间间隔内,堆芯没有有效调节中子毒物数量的方法。幸好,堆芯链式裂变反应产生的部分中子来自裂变产物原子核。某些放射性裂变产物原子核有一个“多余”的中子,但该原子核消除这个“多余”中子的方式不是通过β衰变将其转化为质子(见《放射性与核反应》,而是以一个较长的半衰期(分钟量级)发射出中子,衰变为新原子核。
正是这些延迟发射的“缓发中子”(与裂变反应中瞬时释放出的中子,即“瞬发中子”相对应),提供了调节堆芯毒物数量所需的时间,如提升或插入控制棒,进而控制反应堆功率。如果没有缓发中子,就无法对链式裂变反应进行控制,也就没有核反应堆。
钚原子核的裂变会产生2.9个中子,比铀U-235产生的2.4个中子多。另一方面,钚裂变中缓发中子的比例为0.21%,比铀U-235的0.64%低,这意味着以钚为核燃料的反应堆更难控制。
3. 核反应堆的发展历程
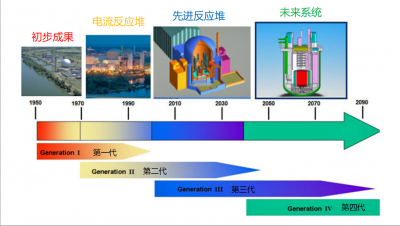
(图中:初步成果—早期原型堆;电流反应堆—商用动力堆;先进反应堆—先进轻水堆;未来系统—下一代核能系统)
自1999年以来,我们一直用第一代、第二代、第三代和第四代来描述核反应堆的发展演化(见图3)。
第一代反应堆指的是20世纪50年代和60年代广泛建设的原型反应堆,它们各有不同,但现在几乎都停止使用了,正处于退役的不同阶段。
第二代反应堆是目前世界上正在运行的主要反应堆系统,提供了世界11%的电力。这些反应堆坚固、可靠、竞争力强。这些反应堆中的绝大多数使用普通的轻水作为冷却剂。
设计建造第三代反应堆的起因是切尔诺贝利核事故,后面我们会具体介绍。鉴于此次核事故的教训,第三代核反应堆的在设计建造时要求,即使在堆芯熔化的极端事故下,放射性仍要能限制在反应堆建筑内部。法国、德国联合设计的欧洲压水堆(EPR)是第三代反应堆的典型代表。EPR是一台功率1650 MW的大功率压水反应堆,装备了多重冗余系统以确保其坚固安全。首先开始建造的两台EPR分别位于芬兰和法国,建造中均遭遇了重大的困难,导致了相当长时间的延期和额外成本。随后建设的两台第三代反应堆位于中国,得益于前两台建造时的经验反馈,这两台在建造过程中并没有遭遇困难。
在退役第一代,运行第二代,建设第三代的同时,反应堆继续发展,第四代堆预计在2050年左右实现商业化运行。
第四代反应堆将面临与今天不同的需求,更好地利用裂变材料,更有效地管理长寿命放射性废料,更好地防止扩散[2],同时安全性至少与第三代一样高,并可以实现多重应用,比如:海水淡化、工业供热以及生产工业氢气用于人工合成燃料等,而不仅仅是发电。
法国超级凤凰堆(RNR Superphénix)是第四代核反应堆的前驱者,从1985年一直运行到了1997年。
第四代核能系统国际论坛(Generation IV International Forum, GIF)汇集了15个具有核技术的国家,给出了6种备选的第四代反应堆堆型,以指导研究和开发。其中某些反应堆将在计划时间内实现工业化。
4. 受控核聚变

当一个重原子核裂变时,分裂前后系统的质量损失会导致相当大的能量释放。类似的(或者说相反的),两个非常轻的原子核融合可导致更大的质量损失和能量释放(就系统的总质量而言)。恒星核心的反应正是这种(图4)。
裂变和聚变都是在1938年发现的,尽管第一个裂变反应堆在1954年就已投入使用,但使用聚变发电在今天看来仍遥不可及。
利用聚变的问题在于发生聚变的轻核都带正电荷,因此相互排斥。在恒星的中心,巨大的引力解决了这个问题,但在地球上,必须找到另一种方法。在氢弹中,是“常规”原子弹的强烈辐射引发了聚变:但这种方式不能用于受控发电。
最有希望实现受控核聚变的方案是利用温度高达1亿度的氘氚等离子体来解决这个问题。氘和氚是两种氢同位素,其原子核分别含有一个或两个中子[3]。氘、氚在这个温度下的运动速度可以克服核间的静电排斥。当然,在这个温度下,这种等离子体不能与任何物质接触:它被一种叫做托卡马克(tokamak)的机器中的电磁场所约束。ITER是这类机器中最强大的,自2005年以来,这台多国合作设计建造的机器一直在法国卡达拉奇(Cadarache)紧张施工。大约在2027年,ITER将会首次实现氘氚等离子体聚变点火:从某种程度上说,这次点火之于受控核聚变的意义,犹如1942年12月费米实验首次实现链式裂变反应之于受控核裂变的意义。
5. 核能与环境
5.1. 温室气体

就环境保护而言,核电的一个主要优势是,在一个完整的生命周期中,核电几乎不会排放温室气体(如图5所示),而温室气体是导致气候变化的重要原因。
5.2. 气态流出物和液体排放
只要不发生事故,核电厂向环境释放的放射性水平非常低,并且受到密切监测。由于燃煤中含有铀和钍等杂质,核电厂释放到大气中的放射性总量比燃煤电厂还低。
核电厂运行产生的强放射性污水,需要通过离子交换树脂来处理特定的污染物。直到各种污染物含量均已达标才允许排放。
5.3. 热排放
无论是核电厂还是其他热电厂,在任何火力发电厂的余热排出口,排出水的温度都比入口处高。这对于建在海边的电厂来说不是问题。但用河水冷却的发电厂,法规规定其温差不能干扰水生动物。因此,这些余热通常需要用空气冷却塔进行冷却后再排放,蒸发的河水产生的羽流从很远的地方就可以看到。在法国,这些冷却塔大多属于核电厂,但在德国通常属于燃煤电厂。
5.4. 放射性废料
放射性极低的核废水和污染物会在稀释后再排放,而放射性废料则会在浓缩后固定在适合其性质的基质进行存储。只要这些放射性废料还存在危险,那么这些固体封装就要在一直储存着。
放射性物质是指含有放射性元素的物质,其数量或浓度要接受监控以预防意外。放射性废料是指没有使用价值或潜在使用价值的放射性物质。在法国,放射性废料的最终处理会在法国核安全局(ASN)的监督下,由法国国家放射性废料管理局(ANDRA)执行。
通常法国对放射性废料会基于两个重要参数进行分类并确定适当的管理方法:一个参数是活度,即废料中所含放射性元素每单位时间的衰变次数;另一个参数是这些放射性元素的放射性半衰期,具体分为放射性半衰期小于31年的放射性废料(即短寿命废料)和放射性半衰期大于31年的放射性废料(即长寿命废料)。
- 半衰期非常短的放射性废料主要来自医疗或研究部门,这些废料的处理方式主要是将其储存在使用场所,直至几乎完全衰变。
- 低于100 Bq/g(花岗岩放射性的20倍)的放射性水平(即活度)非常低的废料(VLW),储存在获得ANDRA正式授权的地表存储场地中。它们主要来自核电厂的废金属、碎石、混凝土,核燃料生产,处理厂和研究中心的运行、维护和拆除工程。
- 长寿命低水平放射性废料(LLW)会受到更严格的管控。这类废料主要是拆除旧核电厂的石墨废料和放射性矿石产生的放射性废料。
- 长寿命的中水平放射性废料(LLW)和高水平废料(HLW)被封装在不锈钢容器中,并且必须被存储在很深的地下。它们主要来自乏燃料后处理。次锕系废料需要进行玻璃固化以包容其衰变产生的热量,然后再进行封装存储。
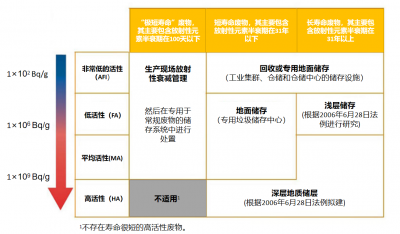
活度水平和半衰期这两个标准,明确了废料分类及相应的管理、处置方案,一种废料对应一种或数种处置方案(见图6)。
乏燃料后处理可以回收铀和钚,同时将所含的少量最危险的废料(MAVL和MA)提取出来以再次处理。这种废料约占乏燃料的5%,经过乏燃料后处理,这种危险废料的体积、产量被压缩。法国每年的产量约为100吨(三辆卡车的容量),有限的产量便于对其进行长期管理和控制。它们被玻璃固化并封装在不锈钢容器中,等待法国工业地质处置中心(CIGEO)深度储存。该中心在2006年得到批准,将于2025年投入使用。
放射性废料的最终管理方面,英国和日本选择了法国和中国的方案,瑞典和芬兰选择了将未经后处理的乏燃料进行地质储存,其他大多数国家的这一问题仍然悬而未决。
6. 核设施事故
6.1. 国际核事故等级重度标度
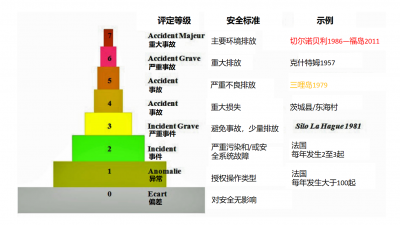
核设施通常很复杂,很难仅从其技术描述中推测事件多么严重。因此,国际核事故等级(INES,International Nuclear Event Scale)得以建立,用以交流影响核设施的事件。INES类似于衡量地震强度的里氏震级,国际核事故等级从偏差到重大事故共分为7级(图7)。
自核时代开始以来,核反应堆发生的事故很少(INES> 3),以下是事故清单:
我们在这里只介绍几个最著名的例子,以及从中吸取的教训。
6.2. 三哩岛(TMI),1979年3月29日
受模棱两可的操作手册误导,这个建于美国三哩岛的反应堆,在操作人员的错误操作下,从一个一般事件演变成了严重事故:三分之一的堆芯熔化,这台几乎崭新的反应堆停止了运行,并花了14年时间进行清理。虽然安全壳经受住了考验,放射性物质的释放很少,也没有人员伤亡,但媒体的大量报道让美国公众受到了持久的创伤。
三哩岛的主要教训是,决定是否安全的不仅是机器质量,还有操作员。世界各地对核反应堆进行了诸多改进,将“人为因素”考虑其中,并分享反馈经验。
6.3. 切尔诺贝利,1986年4月28日
RBMK是苏联设计的一种特殊类型的反应堆,它不仅可以发电,还可以生产用于核武器的钚。RBMK在某些物理条件下会出现运行不稳定的状况。为了进行安全实验,操作人员故意违反了一系列指令,导致链式反应失控,堆芯功率突然增加至具有强大破坏力的水平。产生的蒸汽爆炸摧毁了四分之一的堆芯,炸飞了大楼的屋顶。暴露在空气中的堆芯石墨被点燃:大火持续了约十天,将挥发性放射性物质送入平流层,这些物质沿着喷射流飞越了整个欧洲,并伴随降水和放射性气溶胶的蔓延污染了多国的领土。
约50名消防队员和操作人员死于严重的急性辐射,数千名儿童因事故释放的放射性碘患上甲状腺癌,其中约20人死亡。在“清理者”[4]和普里皮亚季(Pripyat)城镇附近的居民中,或有多达4000人死于辐射诱发的癌症,后来的疏散行动对人们健康造成的损害要更大。
切尔诺贝利的主要教训是,这种污染是无法忍受的。于是,反应堆设计者推出了所谓的第三代电厂(EPR、AP1000等),并遵循一个非常严格的标准:即使是堆芯熔毁也无需长期疏散周边人口。
6.4. 福岛,2011年3月11日
前两起事故主要是人为因素,福岛事故的起因却是自然灾害。一场罕见的9级地震及引发的强大海啸摧毁了日本主要岛屿本州岛的东北海岸,造成20000人死亡。14座核反应堆正分布在受灾地区的4个地点,包括福岛第一核电站的6座核反应堆。地震导致反应堆自动关闭,但也摧毁了福岛第一核电站与高压电网之间的连接。13台应急柴油发动机按计划立即启动,为核电站的反应堆备用系统供电。但是海啸淹没了其中12台,也淹没了应急电板和电池,导致堆芯的衰变余热无法释放(见第1节)。
地震期间,福岛第一核电站运行的三个反应堆堆芯过热,燃料鞘与水蒸气反应产生大量氢气,引发爆炸,堆芯熔化。第四个停堆反应堆也遭到损坏。
第一批释放到大气中的放射性物质被吹向太平洋,在那里被稀释,但3月15日晚上,风向改变了,该地西北约50公里长的走廊受到严重污染。
由于早期的疏散措施,当地居民受到的辐射不大:我们预计其对健康的影响不会大幅高于环境本底辐射。不幸的是,疏散造成了病人或老人的死亡。
福岛事故验证了大多数第三代反应堆的安全性,也证明了以前反应堆的脆弱性:加强备用系统稳定性的新措施正在实施,危机管理系统也在改进(例如,法国电力公司创建了快速行动部队(FARN),能够在事故期间支持甚至替换操作员)。
福岛事故也教会了我们许多关于地表净化的知识,以便疏散的人口重归故土。
7. 放射性污染的治理与自然衰减
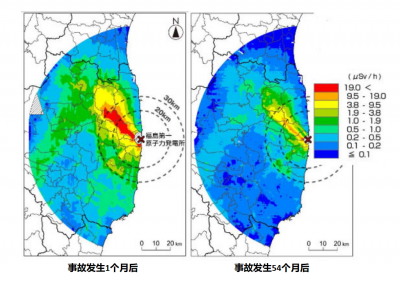
放射性元素会自发分解:经过十个半衰期后[5],活度将降为原有的千分之一(210 = 1024)。因此,核事故发生的前两到三个月,放射性碘会导致甲状腺癌。之后,其影响就可以忽略不计,而铯同位素Cs-134(半衰期约4年)和Cs-137(半衰期约30年)将成为污染的主要来源。
土壤的性质在切尔诺贝利事故污染的地区也起着至关重要的作用:铯很快渗入粘土中,其表面放射性会因此消失,但它实际上一直存在于土壤中。在乌克兰或白俄罗斯的村庄,人们已经了解要避开某些“高危”地区,而在这些地区之外生活是安全的。
事故发生29年后,切尔诺贝利周围的疏散区仍禁止人们进入,日本却在尽一切可能让居民尽快返回福岛。一些地方在事故发生四年后重新开放。他们实施了许多去除核污染的方法:
- 屋顶清洁
- 刮掉地表以上5厘米的土
- 深耕(> 30厘米)
- 利用植物修复
- 住宅区清洁
这些方法的有效性得到了验证,但是会产生大量要储存的放射性废料。森林仍然很难净化,但没有人一直呆在那里。对自然生物多样性的影响似乎是有限的,并且对不同物种的影响也不同[6],辐射的危害也可能通过人类减少在污染区域活动所带来的正向作用抵消。
参考资料及说明
封面图片:圣阿尔邦(Saint Alban)发电厂 [来源:Y. 杰弗雷(Y. Geoffray)提供的阿海珐集团文件]
[2] 降低从民用转为军用的风险。
[3] “等离子体”和“同位素”在《放射性与核反应》一文中有解释。
[4] 该名称特指在现场进行干预,为整个工厂建造防护外壳以净化现场的施工人员。
[5] 每一个放射性原子都有其自己的“半衰期”特点,在半衰期中,原子衰变为原来的一半。
[6] http://www.irsn.fr/FR/connaissances/Installations_nucleaires/Les-accidents-nucleaires/accident-fukushima-2011/fukushima-2016/Documents/IRSN_Fukushima-Tchernobyl_faune-flore_201603.pdf.
环境百科全书由环境和能源百科全书协会出版 (www.a3e.fr),该协会与格勒诺布尔阿尔卑斯大学和格勒诺布尔INP有合同关系,并由法国科学院赞助。
引用这篇文章: BARRÉ Bertrand (2024年4月23日), 核能利用, 环境百科全书,咨询于 2025年4月6日 [在线ISSN 2555-0950]网址: https://www.encyclopedie-environnement.org/zh/physique-zh/controlling-nuclear-energy/.
环境百科全书中的文章是根据知识共享BY-NC-SA许可条款提供的,该许可授权复制的条件是:引用来源,不作商业使用,共享相同的初始条件,并且在每次重复使用或分发时复制知识共享BY-NC-SA许可声明。