Radioactivity and nuclear reactions
PDF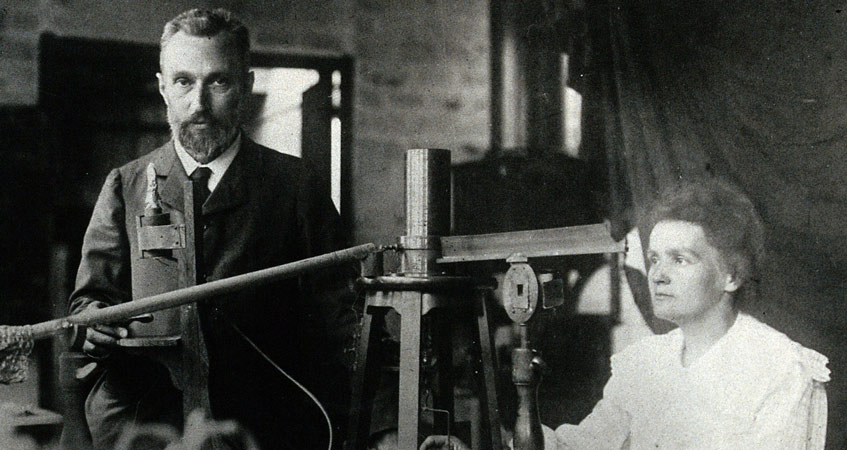
For the nucleus of an atom to be stable, it needs a certain proportion of neutrons and protons. Otherwise, it undergoes a series of disintegrations to reach a stable state by removing excess particles. In doing so, it emits radiation that, in high doses, attacks unprotected living tissue. Each radioactive nucleus is characterized by the nature and energy of the radiation it emits and its specific rate of decay.
1. From stable to radioactive nuclei
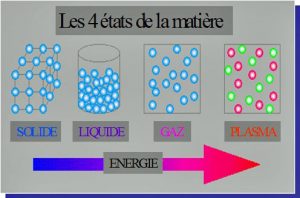
The nucleus is an assembly of massive particles, the nucleons: the mass of a nucleon is more than 1800 times the mass of an electron. Nucleons are divided between protons that carry a positive electrical charge and neutrons that are not charged. The number of protons in a nucleus determines its chemical species (1 proton = hydrogen, 2 = helium, 6 = carbon, etc.). It is the atomic number, denoted Z, while the total number of nucleons or atomic mass is denoted A. The atoms of the same Z whose numbers A differ are isotopes of the same element. Their chemical properties (linked to protons) are almost identical, but their nuclear properties can be very different. Isotopes are commonly noted by their chemical symbol preceded by their atomic mass by exposing: 1H, 4He, 12C, 16O, 235U.
The electrons are distributed over layers more or less distant from the nucleus. Peripheral electrons play a major role in chemical reactions. In a molecule, atoms more or less share these peripheral electrons. An atom has the same number of protons and electrons: it is electrically neutral. If the two numbers differ, the atom is said to be ionized – but it retains the chemical nature determined by its protons. If it has an excess of electrons, it is a negative ion, or anion (example: Cl-, O–), otherwise it is a positive ion, or cation (example H+, Ca++).
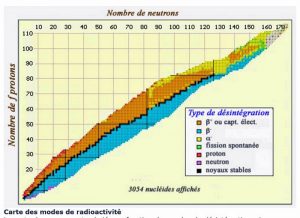
In the nucleus, neutrons ensure the cohesion of protons that would otherwise repel each other, since they have the same electrical charge. The more nucleons in a nucleus, the more neutrons must exceed the number of protons in order for the assembly to remain stable: thus, the nuclei of atoms present in nature are located in what is called the “valley of stability” on a diagram comparing the number of protons and the number of neutrons in the nuclei (Figure 2).
If a nucleus has too many or too few neutrons, it is unstable, and will tend to reach, more or less quickly, the valley of stability, disintegrating according to one of the mechanisms described in the next chapter. This disintegration is accompanied by an emission of energy and radiation: this is called radioactivity.
Natural radioactivity is often opposed to artificial radioactivity: it is an abuse of language, there is only radioactivity, but it can come from nuclei naturally present on Earth (and in particular in the human body which naturally contains potassium 40K, and carbon 14C or nuclei artificially manufactured in particle accelerators or nuclear reactors. Natural radioactive elements can be derived directly from nucleosynthesis in stars and supernovæs, such as potassium 40K and uranium 238U. They can also be formed by the interaction of pre-existing nuclei with cosmic radiation particles, such as carbon 14C. Finally, they may come from the decay of another radioactive nucleus, such as radium, which descends from uranium.
Each radioactive isotope – also known as radionuclide – is characterized by the rate of its decay. This is quantified by the period, sometimes called half-life, denoted T½ , the duration after which exactly half of a given number of nuclei will have disintegrated. The period is a statistical feature: it is impossible to predict when an individual radioactive nucleus will disintegrate, but if we start from one billion of these nuclei, we know with extreme precision when only 500 million will remain. And this characteristic period of a specific nucleus can range from a fraction of a millisecond to a few billion years… (see Table 1).
Table 1: A few radioactive atoms (N=natural, A=artificial)
In the media, the period is often referred to as “lifetime” and associated comments suggest that the longer the lifetime, the more dangerous a radioactive atom is… It is exactly the opposite: if the period is long, it is because the rate of disintegration is slow, and therefore the activity is low!
2. Radiation, activity, doses
A radioactive atom tends to return to the valley of stability by emitting radiation. This can happen in different ways as shown in Figure 3.
2.1. ALPHA radiation (α)
If its nucleus is unstable because it has an excess of nucleons, which is the case for many heavy nuclei, the radioactive atom will disintegrate by ejecting an assembly of two protons and two neutrons, known as a particle α and which constitutes a helium nucleus. In doing so, the atom decreases by two atomic numbers and its atomic mass decreases by 4: plutonium 239Pu thus becomes uranium 235U and uranium 235U becomes thorium 231Th, for example.
2.2. BETA- radiation (β-)
If the radioactive atom is unstable because its nucleus has too many neutrons, a neutron will transform itself into a proton, by ejecting an electron, to keep the electrical balance, as well as a tiny particle called antineutrino (noted ). With unchanged atomic mass, the atom gains an atomic number. Thus iodine 135I becomes xenon 135Xe or neptunium 239Np becomes plutonium 239Pu: it is the radioactivity β-.
2.3. BETA+ radiation (β+)
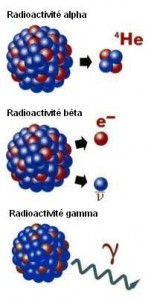
2.4. GAMMA radiation (γ)
Even with the correct number of nucleons, a nucleus can be excited and have to get rid of an energy excess: it does so by emitting very short wavelength electromagnetic radiation, a photon γ (Read “The colours of the sky “). A disintegration β is often associated with radiation γ.
There are rarer forms of radioactivity, corresponding, for example, to the emission of a neutron (see article “Harnessing nuclear energy“, section “delayed neutrons”).
After disintegration, the resulting atom can also be unstable and disintegrate: a “chain” of disintegrations occurs that only stops when stability is achieved. Thus, after 16 successive disintegrations, uranium 238U becomes 208Pb lead, which is stable.
2.5. Units for radioactivity
The activity of a radioactive atom is the rate of its disintegration. It is expressed in becquerel (Bq): 1 Bq = 1 disintegration per second. The becquerel is a very small unit: the natural radioactivity of the human body, mentioned in the previous chapter, is about 8000 Bq. Not enough to require wearing a lead coat to avoid irradiating your neighbours! This is why the activity of a radioactive source is often expressed in giga (billions) or even terabecquerels (thousand billion), which are GBq and TBq. The activity is a measure of rhythm, but not a measure of effect: if you are bombarded at the same rhythm with ping-pong balls or petanque balls, it will not have the same effect! Hence the notion of dose.
When radiation hits a material, it gradually loses all or part of its energy, which is absorbed by the material. The amount of energy absorbed by a given mass of matter is called the dose. The dose is measured in gray (Gy): 1 Gy = 1 joule/kg. Unlike the becquerel, the gray is a very large unit, of which the microgray (millionth) or milligray (thousandth of a Gray) submultiples are mainly used.
In living tissues (see below), radiation damage is not only dose dependent, but also depends on the nature of the radiation involved, and the nature of the tissue irradiated. If the dose is a physical quantity, the equivalent dose is a biological quantity that takes into account these additional parameters by multiplying the number of grays by the appropriate coefficients. It is expressed in sievert (Sv), more often in milli- or microsieverts (mSv, μSv).
The dose rate measures the absorbed dose per unit of time (examples: μSv/h, mSv/year, etc.)
3. The effects of radiation on living organisms
3.1. how to protect yourself from it
Radioactivity is frightening because radiation is not perceptible to our senses – it is invisible, odourless and silent – and because high doses can cause cancer.
If radiation is indeed beyond our senses, it is, on the other hand, easily detectable at minute doses: when we perform a “PET” examination[2], we record each individual disintegration! By comparison, none of our instruments could detect less than a few billion billion molecules of a chemical poison !
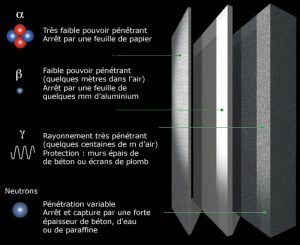
- A sheet of cigarette paper, the small layer of dead cells in our epidermis is enough to stop alpha rays.
- A few millimetres of aluminium sheet can block beta rays.
- 1.5 meters of concrete, or 5 meters of water will stop gammas and neutrons.
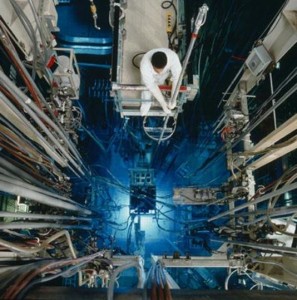
3.2. biological effects of radiation
Because of their high energy, radiation from radioactivity is able to ionize atoms (extract electrons) when they enter matter. This is why they are classified as “ionizing radiation“, as opposed to visible light and longer wavelength electromagnetic radiation (see “The Colours of the Sky“). This ionization can break molecules and cause chemical reactions. Cosmic rays, energetic particles emitted by the Sun or from more distant sources, are also ionizing radiations, as well as X-rays used in medical imaging.
Most of the molecules in our body are constantly regenerated and such effects are then reversible. However, the DNA of our cells plays a particular role in carrying the memory of our genetic heritage, and its preservation is therefore essential. DNA is a huge molecule with billions of atoms arranged on two strands wrapped in a double helix: ionizing radiation can cause damage by damaging one or, worse, both strands at the same time.
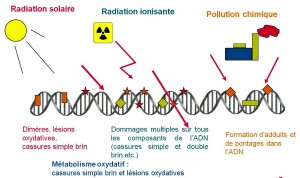
In other words, our repair mechanisms are extremely efficient and fast. In comparison, the natural radioactivity in which we are immersed causes about a few attacks per cell per year.
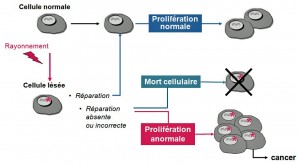
We can see that it is possible for a single irradiation to cause cancer, but the probability is extremely low.
3.3. International radiation protection rules
Created in 1955, the United Nations Scientific Committee on the Effects of Atomic Radiation (UNSCEAR) periodically prepares syntheses of all international scientific data in its field of competence [4]. Since its inception, UNSCEAR has carried out epidemiological studies on “cohorts”, groups of individuals who have suffered significant radiation or contamination: survivors of Hiroshima and Nagasaki, workers of the Soviet Mayak complex, populations living along the Tetcha River, neighbours of the Semipalatinsk airborne atomic explosion site, American radiology personnel, aircrew (exposed to cosmic radiation during their flights), etc. It is on the basis of these studies that the rules on radiation protection are established, i.e. the protection of the public and workers against radioactivity. In summary, the effects of radiation are primarily a function of the dose received, but also of the “dose rate” that characterizes the intensity of that dose.
3.4. High doses
- If a person receives high doses (above 1 Sv), there are many cellular deaths and the effects are visible: lesions appear on the skin, blood, intestine… these lesions are all the more serious when the dose received is high. We speak of a deterministic effect, and of “acute irradiation syndrome”.
- In addition, if a group of people receives high doses, more cancers appear in this group of people than if it had not been irradiated. The frequency varies with the dose, but the severity does not depend on it. This effect, which varies from one individual to another, is called “stochastic“, synonymous with randomness.
3.5. Low doses
While the damage created by high doses is obvious, the low dose range is still unclear. On the one hand, cancers have many sources other than ionizing radiation, and on the other hand low doses are lost in the background noise of variations in natural radioactivity. Moreover, it is very difficult to find cohorts whose individuals differ only in the dose received: for example, very significant doses would be required to bring the cancer risk of a non-smoker to the level of the risk of a smoker! Nevertheless, we can say this:
- Below 100 mSv (0.1 Sv) no observation of an increase in the number of cancers was observed, but if the irradiated and observed populations were larger, it might have been possible to observe some.
- This is why the International Commission on Radiological Protection ICRP, in order to determine dose limits, extrapolates linearly to low doses what is observed at high doses. This assumption of a “linear relationship without threshold” is conservative and used according to the precautionary principle to set radiation protection rules. It may lead to overestimation of the average incidence by ignoring possible threshold effects: the repair mechanisms mentioned above may be more effective at low doses but “saturated” at high doses. In addition, the collective dose in a group of people can cover large individual variations, and the number of cancers appearing presents random fluctuations inherent in such rare events: this number approaches the average only beyond about a hundred cases.
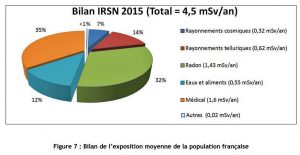
In comparison, the dose due to natural radiation in France varies between 2.4 and 10 mSv/year, and in some regions of the world with particularly radioactive soil, the natural dose can exceed 100 mSv/year. The average French person also receives a dose of 1 mSv/year in medical and dental radiographs. Figure 8 shows the average irradiation of a resident of the Paris basin.
3.6. Contamination
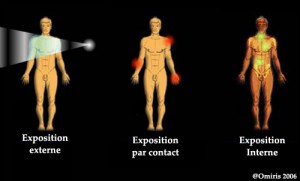
Internal contamination occurs in three ways: by inhalation of radioactive substances or dust; by ingestion, when they are contained in food absorbed or placed on an object placed in the mouth; by injury with a contaminated object or when they stain an existing wound. It is very difficult [5] to decontaminate a person who has been internally contaminated. However, its effect can sometimes be lessened, for example in the case of tritium, by drinking a lot of water.
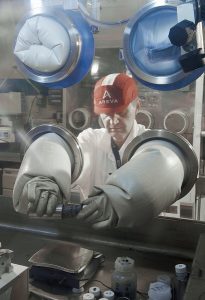
For example, the manufacture of plutonium fuel follows the same steps as that of enriched uranium fuel (very weakly radioactive, read “Controlling nuclear energy“), but operations must be carried out in sealed enclosures called glove boxes (see photo Figure 10).
We cannot close this chapter without recalling the medical applications of radiation, at very high doses: radiotherapy and brachytherapy. Radiation has cured many more cancers than it has caused.
References and notes
Cover image. Pierre and Marie Curie, winners with Henri Becquerel of the 1903 Nobel Prize in Physics for the discovery of radioactivity.
[1] There is a fourth state of matter: plasma, which constitutes 99% of the universe. In plasma, there are no atoms: the nuclei “swim” in a sea of electrons.
[2] Positon Electron Tomography (Positon Electron Tomography) medical imaging, which allows, for example, to show the areas of the brain activated by a given activity.
[3] For example, in the formation of the hand, it is not the fingers that grow, but the spaces between the fingers that kill themselves..
[4] A summary in French of the UNSCEAR report can be found in the IRSN report 2006-74
[5] It is possible, for example, to remove inhaled plutonium particles by washing the lung with a chelating agent (special molecule in the shape of a pliers), but this is a very cumbersome procedure.
The Encyclopedia of the Environment by the Association des Encyclopédies de l'Environnement et de l'Énergie (www.a3e.fr), contractually linked to the University of Grenoble Alpes and Grenoble INP, and sponsored by the French Academy of Sciences.
To cite this article: BARRÉ Bertrand (January 5, 2025), Radioactivity and nuclear reactions, Encyclopedia of the Environment, Accessed April 6, 2025 [online ISSN 2555-0950] url : https://www.encyclopedie-environnement.org/en/physics/radioactivity-and-nuclear-reactions-2/.
The articles in the Encyclopedia of the Environment are made available under the terms of the Creative Commons BY-NC-SA license, which authorizes reproduction subject to: citing the source, not making commercial use of them, sharing identical initial conditions, reproducing at each reuse or distribution the mention of this Creative Commons BY-NC-SA license.