Drinking water production, a major public health issue
PDF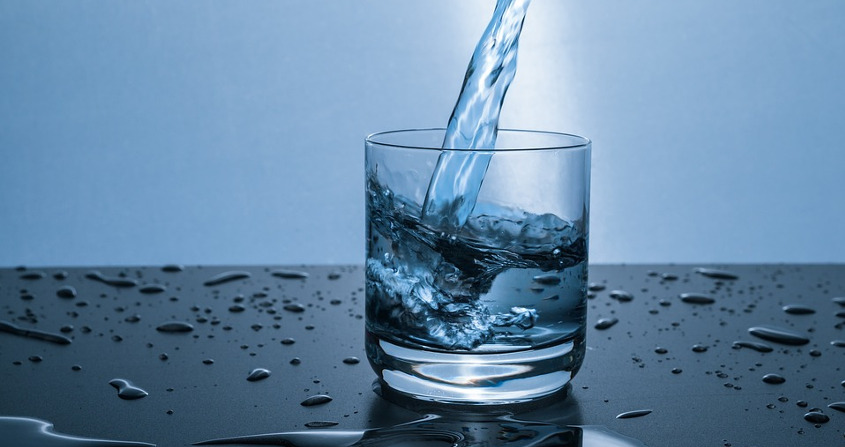
Drinking water supply is a key public health issue. Considerable progress has been made since the end of the 19th century, from the discovery of microbiology to the analysis of ultra-chemical traces. It is one of the main causes of improving public health. On average, each French person consumes 150 litres of drinking water per day, but the volume withdrawn at source is 50% higher. After a section devoted to drinking water production techniques, the regulations are summarized as well as the risks involved, despite fairly thorough but incomplete controls at the microbiological (e.g. cryptosporydium, cyanotoxins, etc.) and chemical (e.g. pesticide metabolites, pharmaceutical residues, etc.) level. The expected impacts of climate change, already observed for some, conclude this article. These include future difficulties in obtaining sufficient quality resources (reduced river flows and aquifer search rates) (pollutant remobilization, less diluted effects).
Water intended for human consumption, also known as drinking water, is defined by regulation as “water that does not contain a number or concentration of microorganisms, parasites or any other substance that constitutes a potential danger to human health and that complies with a certain number of quality limits and references”. It is therefore generally very good quality water that every French person in the residential sector consumes on average 150 litres per day, only 10% of which is used for drinking and cooking. The corresponding groundwater and surface water withdrawals represent 18% of total national requirements, or nearly 42% if we exclude withdrawals for electricity production. The stakes in this area are therefore very high and worrying, given the expected impacts of climate change on the availability of resources but also on their quality.
1. Drinking water supply: a key public health issue
1.1. The discovery of pathogens
The awareness of the health risk associated with water follows the establishment of the relationship between the major European epidemics of cholera and typhoid fever and sewage pollution. In France, these observations led to the 1st ministerial circular in 1900, which announced that a chemical analysis (very simplified at the time) was not enough to assess water safety but that a microbiological analysis was necessary, without specifying the methods. The first elaboration of a regulation will lead in 1962 to an essential but difficult to verify requirement: “the absence of any pathogenic germ”. In terms of control, it is the absence of certain so-called “test” germs that was considered representative of a very low risk of the feared presence of well-known pathogens, such as Salmonella and Shigella.
This health objective of “absence of test germs” has now proved its effectiveness. He is probably responsible for some of the enormous progress in public health in the 20th century. It is indeed the benefit of water filtration that is highlighted since the end of the 19th century, at the time of the major epidemics. It was in the first quarter of the 20th century that the processes of slow filtration and disinfection (bleaching, ozonation, iodization) developed, then clarification using coagulation consists in rapidly mixing the water to be treated with one (or more) chemical reagent(s) to destabilize the colloidal (very fine) particles of the water, and then agglomerating them under slow agitation (flocculation, coagulation), in order to decant (or filter) them more easily. In the 1960s and 1970s, water treatment for drinking water production was still very simplified. Groundwater is simply pumped and chlorinated (or “bleached”) and surface water treatment is designed according to the classic “frequent pre-chlorination, then clarification (coagulation, flocculation, settling, sand filtration) and finally disinfection by chlorination” scheme.
1.2. Continuous progress in the detection of toxic molecules
In recent decades, many chemical molecules have been detected and quantified in natural waters and advances in toxicology and epidemiology have made it possible to assess their health effects. Most of these new substances are the result of increased production and use of natural derived compounds (fertilizers, metals, etc.), as well as new synthetic molecules (pesticides, pharmaceuticals, cosmetics, etc.). Some of these pollutants have long existed in water intended for human consumption, sometimes at higher concentrations than today. However, they have not always been taken into account by past regulations, either because they are considered safe because of their unknown toxicity or, for many, because they cannot be analysed at the concentrations present (e.g. Trihalomethanes (THMs): by-products of disinfection with chlorine gas or bleach, resulting from chemical reactions between the oxidant “chlorine” and dissolved organic matter present (naturally or not) in water, bromates, material monomers, natural hormones, etc.) For most of them, the risk is mainly long-term and regulations are now established in this sense with the notion of the maximum permissible concentration to be respected.
In the absence of very effective prevention and as the risk is never zero, treatment systems must nowadays be adapted, from their design or during their rehabilitation. The first simple processes, mentioned above, have not been sufficient for a long time and now include specific treatment procedures that specifically eliminate a chemical substance present in water (iron, manganese, nitrates, etc.) (rather for groundwater) and/or refining treatments (generally for surface water) to eliminate present or accidental pollution.
Thus, many advanced processes have emerged in recent decades (see § 3). Those using membranes, initiated in the 1980s, are certainly the most important technological leap forward in the field of drinking water production. Will it make it possible to achieve the concept of “chlorine-free water”, which has been sought since the 1990s? This is still unimaginable in the vast majority of cases today, given the need to distribute water in networks that are still very sensitive to the development of microbiological pollution.
2. What drinking water needs and from what resources?
About 10% (0.7 billion) of the world's inhabitants do not have access to drinking water of sufficient quality (there were more than 3 billion in the late 1990s). The demand for drinking water in the world will be increasingly important with the increase in population and global warming, a considerable challenge, especially for countries with less than 1,000 m3 per capita of renewable fresh water [1] (see Risk of water shortage?). France has 2,600 m3 of renewable fresh water per inhabitant, with wide geographical and seasonal disparities. This corresponds to a total of 170 to 175 billion m3, of which 5.4 billion m3 (5.4 km3) are withdrawn for human consumption, slightly less than 2/3 of which is groundwater and the rest surface water.
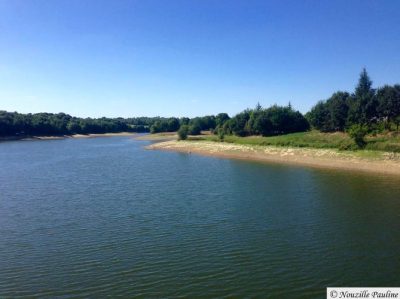
This 5.4 km3 withdrawal to produce drinking water for 67 million inhabitants corresponds to an average of 80.6 m3 per capita per year (or 221 litres per day). In 2012, the average consumption is 54 m3/inhab/year. It is higher in urban areas than in rural areas. This average consumption, which was higher in the recent past, gradually decreased from the 1990s onwards, mainly because of a concern to reduce water bills, but also because of changes in behaviour. The difference between resource extraction and domestic consumption (at the consumer's tap) has several origins. The water loss in distribution system, a set of elements (pipes, tanks,…) used to distribute drinking water between the factory outlet (pumped water) and the consumer's tap (distributed water), relatively constant for about fifteen years, is estimated at about 15.6 m3 per inhabitant and per year. Other losses (in the production plant), consumption by the tertiary sector, the public sector and the industrial sector (consuming drinking water) explain the remaining difference.
What will become of this consumption in the coming decades? This is difficult to predict until climate change adaptation plans are defined and implemented. Some studies [2] predict an increase in consumption of around 1.5% to 2% per degree of increase in the average temperature of France as a result of climate change. However, these figures seem low compared to the increases in consumption recorded during the “heat wave” of June 2017, for example in New Aquitaine. Accommodation measures should limit such increases.
The quality of the resources used to produce drinking water varies greatly depending on the types of substances present (natural and anthropogenic; see table). We can indeed find there:
- biological impurities, such as bacteria, viruses, parasitic protozoa and phytoplankton;
- mineral impurities with no appreciable effect on health or with an indirect effect, such as turbidity, colour, mineralisation, certain transition metals, ammonium and certain dissolved gases;
- mineral impurities with significant health effects, such as heavy metals, oxidized forms of nitrogen, antimony, arsenic, barium, boron, fluorine and selenium;
- organic impurities measured by global parameters : total organic carbon measuring all carbonaceous organic matter present in water by thermal or photochemical oxidation, permanganate index (oxidizability to potassium permanganate) measuring a large part of the carbonaceous organic matter present in water, by chemical hot oxidation;
- proven organic micropollutants (pesticides, chlorinated solvents, hydrocarbons, polychlorinated biphenyls, detergents) and emerging micropollutants (endocrine disrupters, pharmaceutical residues, comethics, etc.);
- impurities of a radioactive nature.
In addition, new impurities are introduced through treatment and distribution, such as residual treatment reagents (aluminum, iron), disinfection by-products (trihalomethanes, bromates, chlorites), lead and monomers of materials in contact with water. The presence of these impurities will lead to different problems to be solved during water treatment.
The main problems encountered during water treatment, due directly or indirectly to the quality of the resource:
3. Drinking water production and distribution in France
In France, the number of abstractions is estimated at about 30,000 (including about 95% in groundwater), but 90% of these abstractions produce only 21% of the volumes. The total volume captured represents an average of 15 million m3 per day (5.4 km3 per year), of which 2/3 is groundwater and 1/3 is surface water (see Karst, a renewable water resource in limestone rocks). About 80% of the surface water resources for drinking water supply are running water (rivers, canals) and 20% of standing water (including 13% of dams and reservoirs). In terms of structures, drinking water supply in France includes about 3,000 plants and stations, several hundred thousand km of pipelines and more than 10 million m3 of reservoir capacity, or an average consumption day.
The establishment of a treatment system (or plant) for the production of drinking water consists in assembling a number of water treatment processes (or unit operations), in a given order, intended to produce pleasant, disinfected water that complies with regulatory requirements (see section 4), while minimizing the formation of treatment by-products. Several technical difficulties are encountered: very rarely excellent quality resources, impact of the network on the quality of the distributed water, increasingly varied processes available, etc… Another difficulty to overcome is the consumer's growing demand for the taste of water, but also his distrust of tap water quality compared to the bottled water he consumes without fear. This mistrust is also maintained by the increasingly prolific media on the subject, with sources that are sometimes erroneous.
The water treatment company must produce water that is tasteless and especially chlorine-free, although compliance with regulations requires that the water be disinfected before and during distribution. The minimization of chlorine taste can only be achieved by applying low doses of this reagent or its derivatives, i.e. on water of excellent quality, which requires effective treatment before final disinfection.
A treatment system for water intended for human consumption must include as a priority an excellent disinfection preceded by three groups of possible treatment steps shown in Figure 2: physical and chemical pre-treatments, clarification, refining treatments.
Pre-treatments are very frequent in surface water treatment. They can be physical at the water intake or on the plant (screening, desanding, settling, de-oiling, microsizing) and chemical (pre-oxidation by ozonation, water disinfection and pollutant oxidation process which consists of injecting a mixture of oxygen and ozone (manufactured in situ) into the water to be treated, remineralization consists in restoring sufficient mineralization to the water (to prevent corrosion in particular) by injecting calcium and carbonates partial for very fresh water). From groundwater, pre-treatment, when available, depends on the particular characteristics of the water. This can be, for example, oxidation (removal of iron, manganese, ammoniacal nitrogen) or simple aeration (for the same applications and for degassing).
The group of steps following pre-treatment is generally implemented for surface waters. It is a complete clarification, with coagulation, flocculation, settling (or flotation) and filtration. For certain low turbidity waters (lake, alluvial groundwater, karst groundwater), a single (or double) filtration with coagulation on filter or a filtration on membrane material or organic sheets or tubes, physically retaining particles and micro-organisms by direct filtration (microfiltration process using membranes capable of retaining, by direct filtration, particles of the micrometer order (cf. membranes), ultrafiltration process using membranes capable of retaining, by direct filtration, particles of the order of several tens of nanometers (see membranes), nanofiltration process using membranes capable of retaining, by direct filtration, dissolved particles and species of the order of a few nanometers (cf. membranes) or separating dissolved species by osmotic phenomenon (reverse osmosis process using membranes capable of retaining, by osmotic phenomenon (inverted) under high (or low) pressure, all (or almost all) dissolved substances from water (cf. membranes) ultrafiltration may be sufficient.
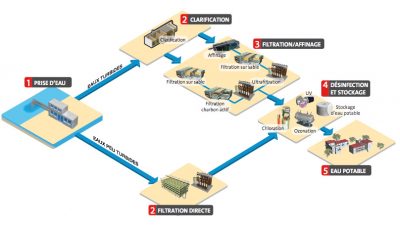
The refining stages have long consisted of filtration on active carbon solids in the form of granules or powders, resulting from the calcination of carbonaceous materials (wood, coal, coconut, etc.), with significant adsorption properties, particularly with regard to organic micropollutants (pesticides for example) in granular form, often preceded by ozonation. Although also used in groundwater, particularly during denitrification or pesticide removal, this refining treatment consists in adding at the end of the traditional water treatment process, one or more additional processes that will refine the quality of the treated water on certain chemical (natural organic matter, micropollutants,…) and microbiological (viruses, parasites,…) parameters. It is rather reserved for surface waters. Traditional surface water treatment units have gradually changed in recent years, at this level, by the introduction of new technologies combining powdered activated carbon (PAC) injection and a liquid/solid separation process (ultrafiltration or high-performance settling tank) or by nanofiltration or even low-pressure reverse osmosis.
4. Are the regulations and controls up to the task? What are the risks?
Guidelines for water for human consumption (or drinking water) are generally established at the global level by the World Health Organization, then taken up at the European level, in the form of guidelines, before being translated and sometimes hardened at the national level by decrees and orders. They are implemented by prefects and regional health agencies (ARS) and ultimately by persons responsible for water production and distribution (PRPDE persons responsible for water production and distribution) and mayors. The underlying objectives are, of course, zero mortality, the lowest possible morbidity and a contribution to public health through water quality. It is sometimes on the basis of epidemiological and toxicological studies and, more often, experiments on animals with extrapolations to humans, that “standards” are established, all with the aim of delivering water that is pleasant to drink.
4.1. Regulations imposed by the European Union
Modern regulations in France are based on decrees dating from 1989 to 1991 (based on the first European directive of July 1980), which have set, in addition to microbiological indicators (“germ tests”), maximum permissible concentrations for many chemical parameters. They also prescribed analytical content associated with sampling frequencies that take into account the nature of the water and the population served by the network.
Today (in 2017), the last European directive of December 1998 is in force, in its form transposed into French law in 2001, then its introduction into the public health code in 2003, supplemented by several decrees and orders regularly published. The scope of these regulations covers all waters which, either as they are or after treatment, are intended for drinking, cooking, food preparation or other domestic uses, whatever their distribution, including “spring waters”, but with the exception of “mineral waters”.
As already specified in the introduction, the regulations define water intended for human consumption as not containing a number or concentration of microorganisms, parasites or any other substances that constitute a potential danger to human health and comply with a certain number of limits and quality references defined by the decree. To meet this objective, a list of consumer tap water quality requirements is specified. It includes about sixty parameters, half in the form of “quality limits”, parameters likely to have immediate or longer-term effects on the health of consumers, and the other half in the form of “quality references”, substances with no direct health impact at concentrations usually found in water but which may indicate a drift in water quality and/or a malfunction of installations.
It should be noted that this list of parameters to be analysed and concentrations to be respected is not sufficiently complete to ensure on its own a total guarantee of absence of chemical risks and absence of pathogenic microorganisms. The regulations lay down other types of obligations, such as technical rules of protection and prevention, administrative procedures, quality control procedures, provisions for informing authorities and consumers, crisis management provisions, etc.
4.2. In addition to regulations, internal control of distributed water
Internal control must be ensured by the persons responsible for water production and distribution (PRPDE), on the performance and quality of the “resource, treatment, distribution” package. One of the main elements to be taken into account when defining a control program is the quality (and its evolution) of raw and treated water ex-factory and, of course, of the water distributed. In addition, the review of facilities at the plant and water system level, the implementation of corrective measures, investigations and studies, and information. This internal control is combined with a control carried out by an official laboratory under the conditions set by the regulations.
From a microbiological point of view, compliance with the parametric values of the regulations (including only 2 “mandatory” test organisms: Escherichia Coli and Enterococci) cannot by itself guarantee complete absence of pathogens. Indeed, there is no obligation of results to testify to the total and permanent absence of “microorganisms, parasites or any other substances that constitute a potential danger to human health “, particularly with regard to an increase in the proportion of populations at risk. In addition, the microbiological health risk threshold (announced as 10-4) is sometimes unachievable by analytical control. For some parameters. This is the case, for example, of the Cryptosporidium parasite, for which compliance with this risk would require the analysis of a sample of several tens of m3.
At the chemical level, the presence of new emerging risks is not yet taken into account by health control (pharmaceutical residues, cosmetics, nanoparticles, etc.). Control is not always frequent enough, especially for small distribution units (less than 5000 inhabitants) for which the regulations require only a limited number of controls (less than once a month). This represents 16 million inhabitants for whom drinking water distribution has nearly 12% of microbiological non-compliance (compared to 4% of non-compliance for the entire French population). In addition, for all units, the verification of non-compliance is preventive for future volumes but only reactive for past volumes. However fast it may be, the “damage is done”. It is therefore necessary to complement the current health control based on the finished product and provided by preventive health risk management methods.
It is therefore becoming necessary to supplement regulatory obligations with obligations of means to ensure consumer protection under the best conditions known to date, through an analysis of health risks. This analysis must include the identification and characterization of the hazard and exposure assessment, risk management through the implementation of technical and organizational means to control it (concept of the “multi-barrier” sector), communication to the various stakeholders, accompanied by an analysis of possible misunderstanding and solutions that can remedy it.
5. The impact of climate change
5.1. On the amount of water available
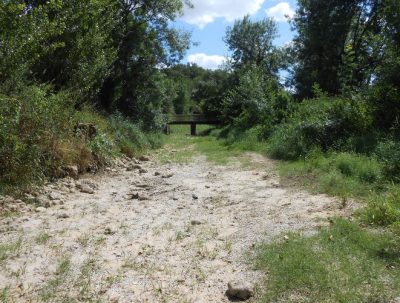
Current scientific evidence [4] shows that climate change, already at work in France, will have significant and alarming consequences on resources for some particularly affected geographical areas. This is the case, for example, in the great southwest of France, for which projections over 2050 predict an average decrease in natural river flows of 20 to 40% and a decrease in groundwater recharge of 30 to 55%. It is therefore possible to envisage scarcity of the available resource, particularly during episodes of extreme heat and drought and, as a result, an increase in conflicts of use and over-consumption of drinking water (see § 2).
5.2. On the quality of the resource
A deterioration in the quality of surface water resources is also feared in periods of drought or heavy and sustained rainfall. The increase in temperature, nutrients and fertilizers, and the corresponding decrease in oxygen, possible salinity intrusions, will inevitably impact microbiological quality. Warming leads to a probable increase in invasive species, often thermophilic and opportunistic, and risks related to endotoxins and parasites.
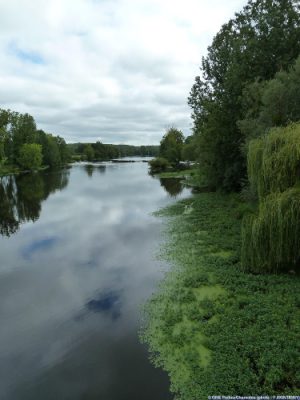
Moreover, at constant quality and quantity of anthropogenic discharges, the effect of less dilution of pollution, coupled with a probable remobilization of pollutants already present in sediments, will lead to an increase in organic and mineral micro-pollution. The question of the quality of the resource for drinking water supply from surface water will also arise, especially when this resource is the only one available. What barriers can be considered to satisfy the likely increase in “raw water” of certain quality parameters such as parasites, cyanotoxins, natural organic carbon (precursor of toxic disinfection by-products), micropollutants, etc.? The development of energy-intensive technologies and the more systematic use of chemical reagents for the treatment of water to be “potabilized” are of course curative solutions to these problems of degraded raw water, but at what costs and with what effects?
What will be the impact of climate change on groundwater quality given that soils still hold significant proportions of fertilizers and plant protection products (and their metabolites) intended to “escape” to groundwater, even more so as a result of temperature increases and extreme hydrological events? Faced with the decline in surface water availability, the significant increase in the population close to the coast and the increase in the demand for drinking water per capita, the shift to groundwater will appear to be a solution. In terms of quality, the major risks of overexploitation will then be amplified transfers of pollutants to deep aquifers and, more locally, probable upwelling of the salt bevel (boundary between seawater and fresh water in coastal aquifers).
5.3. An essential adaptation plan
Whether for surface water or groundwater, a climate change adaptation plan is essential to address the highly likely deterioration in natural water quality. This plan will mainly consist of increasing the level of collection and purification of discharges, limiting non-point pollution and optimising discharges to compensate for the quantitative decline in resources. It must be implemented quickly under the risk of complicating the production of drinking water and harming the natural environment.
References and notes
Cover image. Public domain.
[1] A WATER (2015). Water for a sustainable world, The United Nation Water Development Report 2015, 120 p.
[2] HERBET C., A. PICHON, B. JEUDI de GRISSAC, S. VAUZELLE, E. PARADES (2009). Lessons from the 2003 heat wave and the years 2007 and 2008 for taking climate change into account in estimating future drinking water needs. The challenges for the SAGE deep groundwater of Gironde. Symposium 193 SHF: “Drafts, droughts, rare heat waves and their impacts on water uses”, Lyon, 7-8 October 2009
[3] Extract from LEGUBE B. (2015). Drinking water production – Treatment systems and processes. Dunod Editeurs, Paris, 414 p. and digital supplement
[4] Examples:
BOURAOUI F., G. VACHAUD, L.Z.X. LI, H. LE TREUT (1999) Evaluation of the impact of climate changes on water storage and groundwater recharge at the watershed scale, Climate Dynamics, 15, p. 153-161.
DAYON G. (2015). Evolution of the continental hydrological cycle in France over the coming decades. Doctorate from the University of Toulouse Paul Sabatier. Thesis supervisors: J. Boé and E. Martin.
MEDDE (2016) EXPLORE 20170 Final Report http://www.gesteau.fr/document/bilan-du-projet-explore-2070-eau-et-changement-climatique
The Encyclopedia of the Environment by the Association des Encyclopédies de l'Environnement et de l'Énergie (www.a3e.fr), contractually linked to the University of Grenoble Alpes and Grenoble INP, and sponsored by the French Academy of Sciences.
To cite this article: LEGUBE Bernard (January 15, 2021), Drinking water production, a major public health issue, Encyclopedia of the Environment, Accessed December 26, 2024 [online ISSN 2555-0950] url : https://www.encyclopedie-environnement.org/en/water/drinking-water-production-a-major-public-health-issue/.
The articles in the Encyclopedia of the Environment are made available under the terms of the Creative Commons BY-NC-SA license, which authorizes reproduction subject to: citing the source, not making commercial use of them, sharing identical initial conditions, reproducing at each reuse or distribution the mention of this Creative Commons BY-NC-SA license.