Groundwater in granitic and metamorphic rocks?
PDF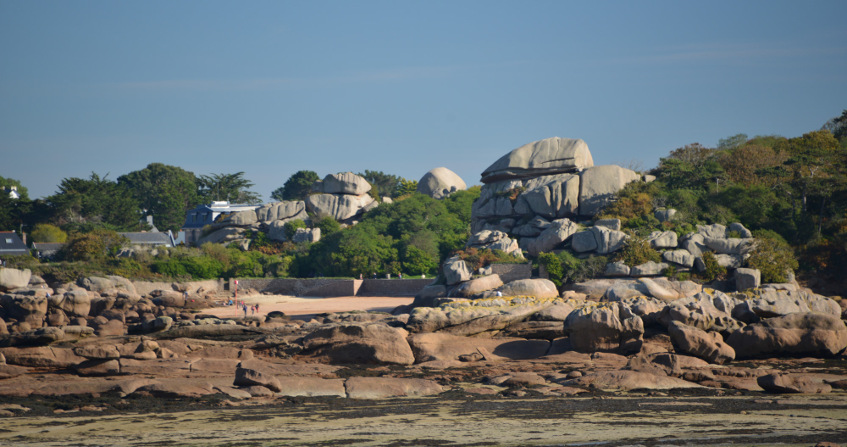
Crystalline rocks or “hard” rocks are generally ancient rocks of two main types: plutonic rocks, such as granites, and metamorphic rocks, such as gneiss, schists, micaschists… They are the foundation of all continents and are therefore very common there. Their hydrogeological properties, although recognized at least since the second half of the 20th century, are now very well characterized, thanks to extensive research conducted over the past 20 years. This article describes how these rocks become aquifers, that is, rocks containing groundwater, and then how hydrogeologists proceed to develop, manage and protect their water resources. A focus is dedicated to the case of India where the groundwater resource of crystalline rocks has made a very significant contribution to the “green revolution”.
1. The water resource of crystalline rocks
1.1. Crystalline rocks
Plutonic rocks, such as granites (Figure 1), are initially derived from magma (molten rock) that has slowly crystallized at depth.
Metamorphic rocks, such as gneiss, schists, micaschists, etc., were formed by transforming (recrystallizing, deforming, etc.) a pre-existing rock (a sedimentary, volcanic, crystalline rock…) under the effect of temperature and/or pressure.
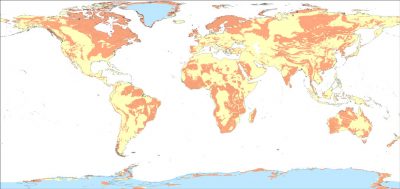
Crystalline rocks were formed mainly in the deep part of the mountain ranges, at a depth of several tens of kilometres, often several hundred million years ago. They were then brought to the surface during the erosion of these landforms. They are therefore the foundation of all continents. They are found outcropping (i.e. near the surface) in many parts of the world (Figure 2 [1]): a very large part of the African, North and South American, Eurasian, two-thirds of India, Australia, etc. In France, they form the so-called “old” massifs: the Armorican Massif, the Central Massif, the Vosges and the Ardennes. They are also well represented in the Pyrenean and Alpine chains, including Corsica, and in several overseas departments or territories (French Guiana, where they constitute almost all the subsoil of this 83,500 km2 department, Saint-Pierre and Miquelon, New Caledonia).
1.2. The challenges and assets of the groundwater resource of crystalline rocks
A rock is aquiferous, i.e. it can contain a groundwater resource, when (1) it has voids (the porosity of the rock), (2) these voids are of sufficient size (at least a few fractions of millimeters thick or diameter) and are connected to each other (the rock is then permeable) and (3) these voids contain water (they are said to be saturated with water), generally provided by precipitation (rain, snow…). This water is then called “groundwater”.
This range of flow available in crystalline aquifers still provides water supply for a population of 400 to 4000 people in a developing country (25 l/d/person) and 50 to 500 people in a developed country (200 l/d/person). Exceptionally enough, the flows used can be very high, several tens of m3/h, thus contributing to the supply of drinking water to cities of several tens of thousands of inhabitants (example of the city of Ploemeur, in Brittany).
This groundwater resource is particularly appreciated in semi-arid or arid basement regions, which generally lack sustainable surface water resources (Sahelian regions, India, Northeast Brazil, etc.). They are often the only water resource available to the population. In regions with surface water, such as Guyana or Equatorial Africa, this groundwater resource is often favoured because of its better quality and therefore lower treatment costs.
… easily accessible and inexpensive.
This productivity, which is generally relatively low, is nevertheless offset by certain advantages.
The water resource of basement aquifers is superficial: less than 100 m deep, often only a few tens of metres deep. The cost of drilling is therefore relatively limited: only a few thousand or even hundreds of euros in developing countries (e.g. India). Drilling technologies very well adapted to this type of rock (mainly the “down the hole hammer” technique; Figure 3), robust and of moderate cost, have been developed in recent decades and are well mastered, both in developed and developing countries.
The water resource of basement aquifers is well distributed geographically; i.e. it is almost always possible to drill a well (Figure 3) near a place of consumption (a village, a field to irrigate, etc.), or to multiply drilling to increase overall production. Thus, for example, in India [2], each rice plot often has its own irrigation well, the size of the plot or plots thus supplied being sized according to the flow available for drilling. Another well supplies the neighbouring plot(s) and so on, within the limit of the overall available water resource, which is directly linked to groundwater recharge by precipitation.
This water resource, which is well distributed geographically, is therefore very “decentralized”. As a result, it does not require heavy investments in water supply pipes. Thus, if it is not adapted for large urban areas, this water resource can supply villages and small towns, even districts of large cities, and allows the development of agriculture (irrigation) and industry.
Finally, due to the low permeability of the subsoil of the crystalline regions, a borehole only influences an extension zone limited to its circumference (several tens of metres to a maximum of a few hundred metres), which is low compared to other types of aquifers. Thus, as soon as a well is at a sufficient distance from a watercourse (several tens or hundreds of metres), it has no significant influence on it. Groundwater exploitation then takes place without impact on the flow of the surface watercourse. This is why, in basement regions, unlike in other types of regions, groundwater exploitation can be carried out with a limited impact on surface water flow.
A water resource that also conditions human settlements.
The modest permeability of these rocks also explains why, in crystalline regions well watered by precipitation (e.g. Brittany, Massif Central), the groundwater surface (or piezometric surface – see Figure 5 – the level from which the rock is completely filled with groundwater) is always very close to the ground surface, at a depth of a few metres at most. Indeed, due to this low permeability, the hydraulic gradient (the “slope” of the groundwater surface) is strong and can therefore have a slope that is close to that of the topographic surface. This is reflected in the presence of springs, albeit of modest flow (often less than one l/s at low water levels), in most topographic depressions (especially small valleys) and in a very dense hydrographic network (streams) fed by groundwater. As a result, traditional habitat can be satisfied in the past with these modest water resources (small springs, streams) and was therefore relatively dispersed. Concentrated habitat, requiring greater water resources, was only possible on the banks of the most important perennial rivers.
In less humid crystalline regions, such as sub-Saharan Africa, the groundwater rarely emerges at the surface of the ground. It is then traditionally captured a few metres deep by large diameter wells, which are relatively easy to dig into the altered, loose part of the rock (see its description in section 2).
Thus, unlike karst regions where traditional habitat is concentrated around karst springs, in crystalline regions it is generally much more dispersed.
The following sections of this article present the hydrogeological properties of crystalline rocks and their consequences in operational terms: location of water wells, management and protection of water resources, etc…
2. How do crystalline rocks become aquifers?
Low permeability rocks..
Intrinsically, crystalline rocks have low porosity and permeability. For granitic rocks, this is because the crystallization of the magma that is at their origin left no space available (porosity) in the rock. For metamorphic rocks, this is because the metamorphic processes have completely obliterated the original porosity and permeability of the original rock, if any. For this reason, these low-permeability rocks are often used to store waste, including radioactive waste [3]. Indeed, water can only pass through them with difficulty and therefore cannot easily carry the pollutants contained in this waste outdoors.
Basement rocks only acquire porosity and permeability when they fracture, that is, when stresses are applied to them and they break. Fractures, i.e. breaks in the rock, then form in their midst (Figure 4). From metric to decametric extension, from a few fractions of millimetres to a few centimetres thick, they give the rock a porosity and permeability and therefore make it “aquiferous”. We speak of “fracture permeability”. These permeability acquisition processes have some similarities with those for limestone rocks. The latter are also generally not inherently permeable. They acquire their permeability not by alteration and fracture as basement rocks, but by dissolution.
Hydrogeologists have long thought [4] that these fractures were caused either by tectonics (the stresses that affect the earth’s crust due to the tectonics of the plates) or “decompression” (the principle is to say that bringing a rock to the surface, due to erosion, reduces the pressure within it due to the decrease in the weight of the overlying land, and would lead to it breaking).
In fact, it has been shown that decompression cannot break rocks under the conditions in which we observe it and that plate tectonics can only be invoked in a few very specific situations but cannot explain all the aquifers in basement regions. However, it has been shown that it is the alteration processes that cause fractures [5], [6], [7].
Indeed, when exposed near the ground surface (a few tens or hundreds of metres from it), rocks deteriorate. Rainwater infiltrates and diffuses very slowly into them, imports and exports ions in solution and thus causes changes in the minerals that make up the rocks. Basement rocks are particularly sensitive to these processes. Some minerals, such as biotite (also known as black mica), abundant in basement rocks, have the property of increasing in volume when they undergo such alteration mineralogical transformations. This increase in volume at the scale of the mineral (a few fractions of millimetres) generates stresses at the scale of the rock which, in turn, lead to the appearance of microfractures and then fractures (Figure 4).
From top to bottom, an alteration profile schematically includes (Figure 5):
* a lateritic or bauxitic armour. Often eroded in metropolitan France, it corresponds to an indurated horizon composed of iron and/or aluminium oxides;

- the saprolite, which can be several tens of metres thick. The original rock is completely transformed by alteration, usually into clay or sandy clay; it is therefore loose (Figure 6). This part of the alteration profile is not very permeable (fractures of the fractured horizon have disappeared, the pores of the rock are small and poorly connected to each other). But it is relatively porous and therefore contains water. Its porosity is between about 1 and 10%, i.e. one cubic metre of rock contains 10 to 100 litres of water; for a thickness of 30 m, there are 300 to 3000 litres of water under each m2. This part of the alteration profile provides groundwater storage;
- the fractured horizon (Cover image, Figure 1, Figure 4). Its thickness also reaches several tens of meters; it is generally twice as thick as saprolite. Only certain minerals in the rock are altered, so the rock remains hard overall. The fractured horizon has many fractures, which are less frequent as depth increases. It is therefore permeable. This part of the alteration profile allows groundwater flow. Wells or boreholes that intersect one or more permeable fractures at this level have exploitable flows that can reach several cubic metres per hour (m3/h). Since the fractured horizon has a low porosity (of fractures) of about 1%, it contains only a few or tens of litres per m2 over its entire thickness. The water pumped from the boreholes comes mainly from the overlying saprolite after a certain time when it is saturated with water.
- more deeply, under the fractured horizon, the healthy rock is impermeable; except sometimes, very locally, where certain discontinuities (old fractures of tectonic origin, veins, contacts between different lithologies…) may have guided the alteration more deeply; in this case, the water pumped there also comes from the underlying fractured horizon and saprolite. Overall, with the exception of these few very specific areas, healthy rock does not contain any groundwater resources
In summary, groundwater in basement aquifers is therefore contained within these two horizons: saprolite and fractured horizon. Basement regions where alteration patterns have not formed or have disappeared due to erosion are poor in groundwater.
3. How to exploit and manage groundwater resources in the basement regions?
Knowing where to drill or drill a well to provide groundwater… Modern methods for detecting and mapping alteration profiles, and therefore characterizing the aquifers they contain, have been developed in recent years, and are applicable at all scales, from the scale of a country, region or department as a whole, to the very local scale of a commune, for example
Which rocks deteriorate best, which are therefore the most fractured? Where is it best to consider drilling? If it is not in the immediate vicinity of the water use, how much will the supply pipes cost?
What is the probability of intersecting a permeable fracture in the cracked horizon? What is the flow rate of a water well that has intersected one or more of these fractures? So how many boreholes must be drilled to meet given needs? And so what will the cost of the project be?
This precise hydrogeological characterization of the subsoil also allows many other practical applications (Figure 8):
- beyond the establishment of water wells, the establishment of their protection perimeters;
- the choice of landfill sites for household waste, on land that meets the legal criteria for impermeability;
- the location of quarry sites, where the thickness of sterile materials to be stripped is minimal;
- the characterization of the impact of tunnels [9], which can dry out surface sources and also whose construction can be hampered by excessive groundwater inflows;
- the consideration of risks of geological origin in land use planning;
- etc.
Despite these modern methods, some uncertainty remains. Some boreholes do intersect fractures, but they may not be permeable.
… but also to know how to manage this water resource..
Once a well, or a set of wells, has been drilled in a given region (or even better, before drilling), it is necessary to ensure that the available water resource is available.
The hydrogeologist of the basement regions then evaluates the groundwater reserve, i.e. the quantity of groundwater contained in the aquifer (of basement) captured by the drilling, mainly in the saprolite. If we draw a parallel with surface water, the reserve is the quantity of water contained in a dam and therefore the quantity available for exploitation.
However, this estimate is not sufficient. It is also necessary to assess groundwater recharge, i.e. how this reserve is renewed and replenished, usually annually, during the rainy season. If we draw a parallel with surface water again, the recharge is the amount of water from precipitation (rain, snow) that will refill the dam once it is partially or totally emptied. In the case of groundwater, it is the amount of precipitated water that will infiltrate the subsoil. In other words, it is the fraction of precipitated water that escapes evaporation and transpiration from plants (often nearly 50% of precipitation in our temperate latitudes) on the one hand, and surface runoff on the other. In the basement regions, the annual share of infiltrated rainfall ranges from a few percent to 20 to 30%.
The available groundwater resource therefore depends on the proportion, and therefore the quantity, of infiltrated precipitation and the surface (or “catchment area”) that will contribute to the supply of the borehole(s) under consideration. Managing groundwater resources by not exploiting any more than its renewable component ensures the sustainability of exploitation. This is referred to as “sustainable management” of water resources. Aquifers therefore contain a water resource that escapes rapid runoff in the rainy season and remains available for use, particularly in the dry season when surface water is no longer available. The groundwater supply available in basement aquifers corresponds to several years, even 15 to 20 years, of average recharge [10]. This reserve therefore makes it possible to cope with periods of drought. Nevertheless, if it is not managed in a sustainable way, i.e. if it is overexploited, it will run out in a few years.
In order to avoid these risks, different methods and tools for resource management have been developed (see in particular the focus on India). Some of these tools, known as numerical models, are designed to simulate the entire water cycle of a given region. They make it possible to assess the available resource in a chosen catchment area and to construct management scenarios and policies, depending on the geological and hydrogeological structure of the subsoil, climatic conditions, water uses, impacts considered acceptable, in particular with regard to reducing the flow of rivers due to groundwater exploitation, etc. These numerical models were first developed for sedimentary aquifers. In France, sedimentary basins have been modelled, such as the Aquitaine basin and the Paris basin for example. The development of the concepts presented above has made it possible to apply similar models for basement aquifers.
… and protect it
The knowledge of the subsoil as described above also makes it possible to map the preferential recharge areas of basement aquifers and to determine in particular the ability of the soil to retain or purify pollutants.
This allows spatial planning policies to be proposed to protect groundwater, for example by avoiding polluting activities in areas where soils have a low purifying power. Effective protection measures, or even measures to restore groundwater quality in areas where it is degraded, can also be implemented.
References and notes :
Cover image. The ,fractured horizon of granites exposed on the surface at Ploumanac’h, Côtes d’Armor, on the pink granite coast [Source : © R. Wyns]
[1] Lachassagne, P., Dewandel, B., Wyns, R. (2014a). Hydrogeology of Hard Rock aquifers. In Hanbbook of Engineering Hydrology. Fundamentals and Applications. Edited by S. Eslamian. CRC Press, Taylor & Francis Group, Chapter 14, pp. 282-310
[2] Dewandel, B., Perrin, J., Ahmed, Sh., Aulong S., Hrkal Z., Lachassagne P., Samad M., Massuel S. 2010. Development of a Tool for managing groundwater resources in semi-arid hard rock regions. Application to a rural watershed in south India. Hydrological Processes. 24, 2784-2797
[3] Storage of nuclear waste in crystalline rocks (Sweden and Finland) http://www.skb.com/, http://www.posiva.fi/en
[4] Lachassagne, P., Wyns, R., Dewandel B., 2011. The fracture permeability of Hard Rock Aquifers is due neither to tectonics, nor to unloading, but to weathering processes. Terra Nova, 23, 145-161,2011
[5] Wyns, R., Gourry, J.-C., Baltassat, J.M., Lebert, F., Multiparameter characterization of subsurface horizons (0-100 m) in altered basement context, PANGEA 31/32: 51-54, 1999
[6] Wyns,R., Baltassat, J.M., Lachassagne, P., Legchenko, A., Vairon, J., Mathieu, F., ñ ñ ñ ñ ñ ñ Application of SNMR soundings for groundwater reserves mapping in weathered basement rocks (Brittany, France) ñ Bulletin de la Société Géologique de France 175, (1), 21-34, 2004
[7] Lachassagne, P., Dewandel, B., Wyns, R. (2014b). The conceptual model of weathered hard rock aquifers and its practical applications, ,in “Fractured Rock Hydrogeology”, N°20 International Association of Hydrogeologists Selected Papers, Editor J. M. Sharp, Jr, CRC Press, Taylor and Francis Group, pp. 13-46
[8] Lachassagne, P., Dewandel, B., Wyns, R. (2014b). The conceptual model of weathered hard rock aquifers and its practical applications, in “Fractured Rock Hydrogeology”, N°20 International Association of Hydrogeologists Selected Papers, Editor J. M. Sharp, Jr, CRC Press, Taylor and Francis Group, pp. 13-46
[9] Dewandel et al., 2017. Dewandel, B., Caballero, Y., Perrin, J., Boisson, A., Dazin, F., Ferrant, S., Chandra, S., Marechal, J.C. 2017. A methodology for regionalizing 3-D effective porosity at watershed scale in crystalline aquifers. Hydrological Processes. 2017;1–19. DOI: 10.1002/hyp.11187
[9] Lachassagne, P., Maréchal, J.C., Bienfait, P., Lacquement, F., Lamotte, Cl. 2014. Computing the water inflows discharge and assessing the impacts of tunnels drilled in Hard Rocks. IAEG XII Congress, Torino 2014. G. Lollino et al (eds.), Engineering Geology for Society and Territory – Volume 3. DOI: 10.1007/978-3-319-09-09054-2_119, © Springer International Publishing Switzerland 2015, pp. 595-599
The Encyclopedia of the Environment by the Association des Encyclopédies de l'Environnement et de l'Énergie (www.a3e.fr), contractually linked to the University of Grenoble Alpes and Grenoble INP, and sponsored by the French Academy of Sciences.
To cite this article: LACHASSAGNE Patrick, DEWANDEL Benoît, WYNS Robert (February 7, 2019), Groundwater in granitic and metamorphic rocks?, Encyclopedia of the Environment, Accessed July 22, 2024 [online ISSN 2555-0950] url : https://www.encyclopedie-environnement.org/en/water/groundwater-in-granitic-and-metamorphic-rocks/.
The articles in the Encyclopedia of the Environment are made available under the terms of the Creative Commons BY-NC-SA license, which authorizes reproduction subject to: citing the source, not making commercial use of them, sharing identical initial conditions, reproducing at each reuse or distribution the mention of this Creative Commons BY-NC-SA license.