水文测验:为什么要测量河流流量,怎么测?
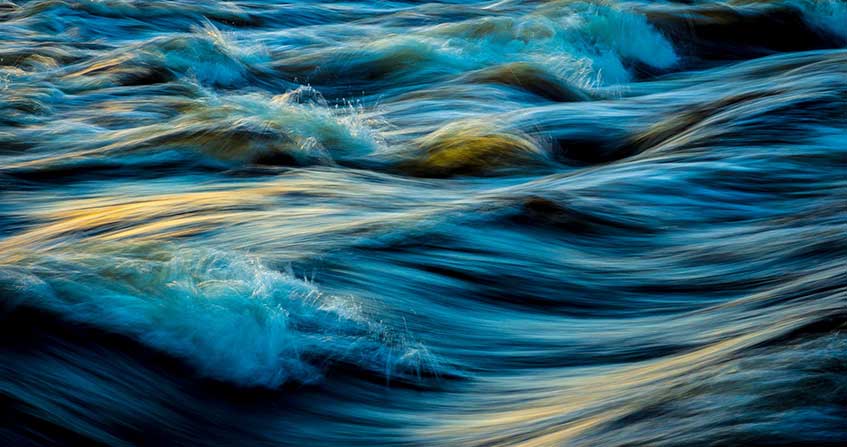
在防洪、供水、农业以及能源生产中,预测和管理河流流量都是必要的,这就需要事先了解如何测量河流流量,由此诞生了水文验测——一门与水文学(研究自然环境中水的科学)和水力学(研究水流的物理学)相辅相成的独特科学。在全球范围内,陆地上的降雨约有三分之一返回海洋(另外三分之二直接蒸发或被植物吸收),每年有近36万亿立方米的水流经河流,但这些水在空间(不同大陆之间、同一河流的不同河段之间)和时间(不同年间、同一年的不同时段之间)上的分布非常不均匀,因此只能通过不断测量这些河流的流量来逼近准确数值。然而,河道流量的连续数据并非简单易得,而是需要综合多种实地测量方法的观测结果。
1. 测量河流流量——一个古老的难题
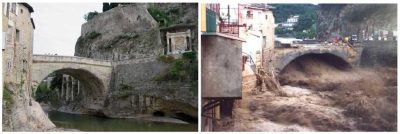
这条地处普罗旺斯的河流的流量波动范围能够从1990年夏天的0.1 立方米/秒(左图)跨越至1992年9月22日的近1200立方米/秒(右图),年平均流量接近6立方米/秒。[来源:EDF DTG]
水文测验主要研究如何测量河流流量。它是一门独特的科学,与水文学(研究自然环境中的水的科学)和水力学(研究水流的物理学)相辅相成。流量是指单位时间内通过过水断面的水的体积,其单位为立方米/秒(m3/s)。
每条河流都有自己独一无二的变化特征,由降水节奏和其水文条件决定。比如世界上最大的河流——亚马孙河,每年两个极端月份的流量值相差只有一到两倍,其河口的年平均流量约为206000立方米/秒,不同年间的变化量仅有10%到15%。可见,亚马逊河是一条非常稳定的河流。
与亚马逊河相反,像沙里河这样的非洲河流在乍得湖出口的平均流量为1197 立方米/秒。同一年内,两个极端月份之间的流量相差可达20倍(由150 立方米/秒变化到3000 立方米/秒),不同年份间的平均流量相差可达两倍,如1942年的年平均流量为739 立方米/秒,1956年则为1720 立方米/秒。因此,沙里河的水文特征变化更加显著。
这些流量是如何测量的呢?自古以来,尤其在人类认识到其与农业的关系时,这个问题就备受关注。但解决这个问题的实际困难程度却超乎想象,以至于让詹姆斯·琼斯(英国物理学家,1877-1946)写下这样一句话:“将单位时间内太阳的总辐射转化为质量,大约是伦敦桥下泰晤士河中流动的水量的10000倍; 不过,即便这个数字并不准确,也不能怪罪于我们无从知晓太阳辐射的确切质量,而只能怪我们无法测量泰晤士河的平均流量。”
2. 为什么要测量河流流量?
河流流量的测量有以下用途:
- 指导水工建筑物的运行管理(水力发电设施、灌溉系统、防洪池或低水位支撑系统等);
- 指导水工建筑物的尺寸设计(基于对水流特征的了解);
- 指导河流流量的管控(确保流量释放量满足下游鱼类存活的最低要求并保障水利设施的其他功能正常,不加剧洪水);
- 保护财产及人员安全(通过判定旱涝灾害情况、发布洪水预警等);
- 帮助掌握河流变化规律(通过一系列的长期观察积累的历史数据,了解河流的演变规律,提高对自然风险的认识,获知旱涝极端事件发生的概率等)。
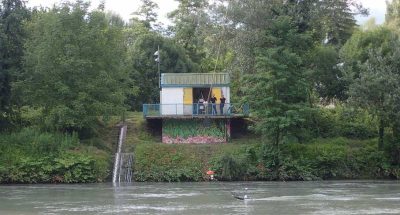
如今人类面临着诸多挑战,如全球变暖、水资源在不同用途(娱乐、能源、灌溉、饮用水)之间的新的需求和合理分配、恢复和保护自然环境及生物多样性、满足社会发展对知识的新需求、社会脆弱性提升等。这些问题也与河流息息相关。在此背景下,对河流流量的测量变得越发重要。
目前,法国大城市约有3500个水文测量站,主要由环境部门和水力发电及灌溉工程的经营者进行管理。其中80%以上的水文测量站使用远程实时传输数据。这样的站点密度(每100平方千米0.63个)是西欧的平均水平,与英国相近,高于西班牙,但低于瑞士和德国。
3. 如何测量河流流量?
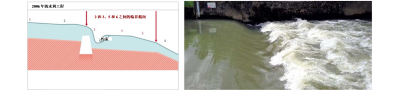
左图为原理图,右图为安装在河中的实例。注意:一旦越过阈值,就会形成一股急流,引发水跃现象,进而产生涡流,造成巨大的能量损失。[来源:© EDF DTG]
直接测量流量的操作非常复杂,除特殊情况外,无法直接连续监测流量。现实中,利用校准曲线建立水位与流量的关系,然后通过连续测量水位推算流量。因此水文测验分为四个步骤:
- 测量选址:选取水力控制设施的上游(见图3)或其他适合连续测量水位高度-流量关系的位置;
- 水位测量:搭建仪器,实现水位的周期性连续测量;
- 流量换算:绘制水位-流量的校准曲线并掌握其变化规律,实现水位与流量的换算;
- 分析归档:对流量的时空波动性进行分析,最后归档。
3.1 水位高度的连续测量
在很长一段时间里,水位测量就是每天(或更频繁)利用刻度尺目视读数(图4)。后来人们利用传感器来监测一个时间步长内的高度变化,其中时间步长是根据流量的波动性确定的(在小型急流盆地中波动较大,在广阔的平原流域波动较小)。目前正在使用的有几代传感器,包括浮标式、气动式、压阻式、超声波式、电导率式等。
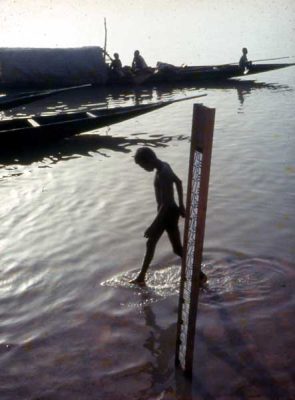
上述设备需设置在水中或水面上,而21世纪初问世的雷达水位计(图5)则可在水面之上工作(设备不受水、沉积物和水中漂浮物的影响,更加耐用),而且对温度不敏感(克服了水下超声的劣势)。然而,需要将雷达移离岸边(边缘效应),而且需要通过波导调节雷达波的接收,这使得雷达水位计的代表性受到一定程度的质疑。
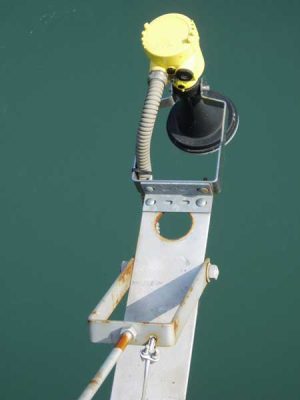
3.2 测量校准曲线的仪器
对河流能够达到的所有流量范围(干旱、中等水流以及洪水)进行的定期测量,主要是通过流场探测或示踪法实现的。
长期以来,流场探测(图6和图7)的测量范围一直局限于水面上(一般通过能够指示水流的棍状装置——“浮子流量计”来测量)。目前,通过速度传感器(机械转子,图6)可以获得更加完整的流场图,其原理是在水流驱动下,螺旋桨的转动与由此产生的感应电压成正比(法拉第定律),即流速与感应电压成正比。
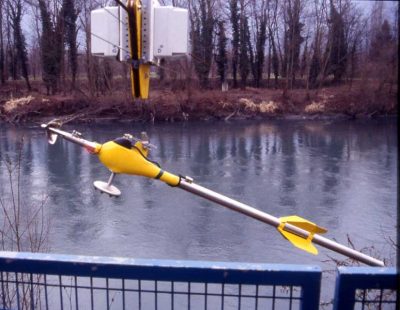
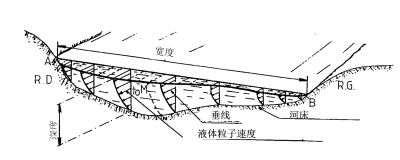
速度分布图是通过对不同深度上的流速进行持续30到40秒的测量而得
20世纪90年代初,声学多普勒流速剖面仪(一种基于多普勒效应的海洋学设备)的诞生,标志着水文测验真正的技术性飞跃(图8)。这项技术大大减少了现场测量所需的时间,尤其适用于较大的河流上,现在也能够用于深度超过50厘米的小型河流。
通过将超声脉冲发射到水中,分析悬浮粒子背向散射回波的频率偏移。该设备通常有3到4个传感器在垂直方向发射发散声束,使其可以测量三维空间中的速度分布。[©EDF DTG]
示踪剂(对动物和植物无害)不会使水变色。投入水中之后会采用荧光计分析其稀释程度(通过测量荧光衰减),不过目前这项工作需要在实验室进行,之后会发展到直接在采样点进行测试稀释程度[©EDF DTG]
示踪法测流(图9)是通过将示踪剂注入到水流中,监测其浓度随时间的变化。在示踪剂充分混合、测量河段内没有水流损失的条件下,根据质量守恒定律,稀释系数与河流流量成正比。历史上曾使用过几代示踪剂,目前倾向于使用荧光示踪剂(如罗丹明和荧光素)或食盐。
3.3 建立水位和流量关系的校准曲线
建立水位与流量之间关系的校准曲线(图10),是流量测量过程中最为精细的环节。长期以来这条曲线由专业人员手工绘制,如今这条曲线的确定也可以依靠决策支持工具,它综合了统计学方法,考虑了仪器测量和水力模型中的计量不确定性。
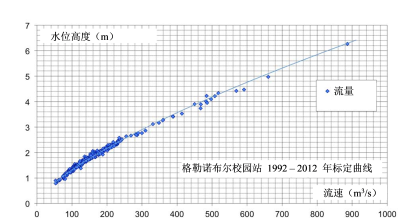
在大多数河流测量站点校准曲线都会因多种原因发生变化,而且它在原则上也永远不会趋于稳定。在格勒诺布尔校园站的例子中,图示的校准曲线在1992到2012年间是有效的。但是随后,由于2012年9月伊泽尔河堤坝工程启动,这一曲线在2013年4月份之前剧烈变化,并在之后再一次趋于平稳。[©LTHE,现为IGE]
水位与流量之间的关系并不会像人们预期的那样稳定,特别是当水力控制不是来自人工构筑物的时候。植被、人类活动、洪水,通过固体运输、侵蚀或沉积等机制,都会或多或少地对河流流量分布产生影响。因此校准曲线的监测需要讲究策略,需要同时考虑测量时间(测量频率)以及水文条件(低水位、平均水位、洪水等)等因素。监测和绘制校准曲线是水文测验的核心工作。
目前已发明出能够连续测量流速的固定式设备,可以在水面上(测速雷达)或在水流中(时差法超声波或多普勒效应)工作。但是水文测验的基本原理从未改变,那就是找到水位、流速和流量之间的校准关系,并在实际测量中进行校准。这些测量设备在高度和流量关系确定之前(在河流中受航行或潮汐影响)就已经问世,而得益于当前的科技进步,它们的安装成本大为降低。
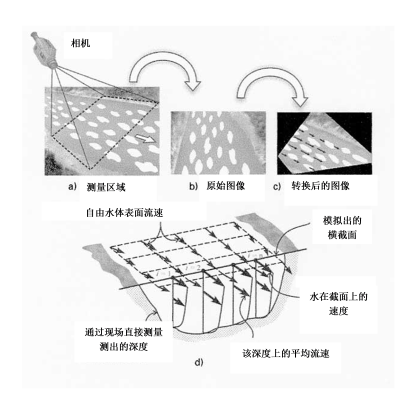
a)漂浮物播种,b)图像记录,c)正射校正,d)流计算LSPIV表面速度测量(来自Muste)
新的流场成像技术在流量测定方面极具应用前景:利用视频图像处理技术,通过水面所有固体的位移(树枝,气泡,树叶……)以及河流的湍动来确定河流表面的速度(图11)。这项技术起源于实验室使用的粒子图像测速技术(Particle Image Velocimetry,PIV),但主要用于大型河流的研究,故称大尺度PIV(LSPIV),其功能包括:(1)记录水流图像的时间序列;(2)对图像进行几何校正以避免形变干扰;(3)使用与模式相关的统计分析方法计算水流示踪物的位移;(4)在已知断面几何形状的情况下,根据垂直流速分布模型,通过LSPIV流场估计总流量。
视频图像处理技术也为未来辅助防洪措施开辟了道路。洪水一般具有突发性,导致道路堵塞(道路被洪水淹没)和安全风险(如汹涌的水流),阻碍抢险队伍的及时干预,而视频图像处理在面对这些因素时可以很好地发挥作用。但是,这项技术也存在限制条件,在能见度低(夜晚、雾天等)的情况下不能使用。
3.4 数据一致性的检查
由水位值导出流量值后,对结果进行评价并将其归档到数据库,是水文测量工作的最终环节。具体包括:对站内的记录进行一致性测试(识别位移和传感器漂移、平滑原始信号、填补缺失记录等);借助不同的水文模型,使本站点与上下游其他测量站点之间的数据规律一致;参考测量地已有的历史数据,结合降雨量及取水等影响因素对测量值进行解释。
整个测量过程是重复进行的,因此可能会发现校准曲线甚至校准策略的问题,从而对其进行进一步的完善。水文事件发生较长时间之后获得的信息(水力建模、洪水测量等)可能与当时的监测结果有极大不同。因此,通常会设置18~24个月的时间用于整编信息。
近年来,水文测验不确定性的量化取得了很大进展,但仍需进一步研究。对于最优的监测站(即校准频率小于每年4到5次),80%的时间内河流流量的不确定性应被控制在5%以内。
4. 水文测验目前面临哪些挑战?
水文测验是一个劳动力密集的作业过程,它要求现场作业,对作业人员在计量、水力学、水文学等方面的专业知识要求较高,是一项具有工匠精神的工作。一个观测站一年的运行成本通常与设置监测点的初始投资成本处于同一量级。因此,水文测量是一项长期任务,削减其预算会对数据质量产生重大影响。
水文测验也是一个复杂的过程,因为它会影响自然环境,牵涉到所有环境相关的危害,数据确认所需的时间可能很长。因此,在信息发布数年之后,测量现场给出的信息(甚至用于决策的信息)和经过考证或发现了新要素之后的综合确认数据之间,可能会有显著的差异(在洪水或干旱等极端情形下,局域极值间差异可能达到两倍之多)。
最后,水文测验也在不断的发展中。新的成像技术(LSPIV)和通信手段的产生(电话、互联网)将大大增加数据量,由此产生了新的问题(比如要如何处理、考证、同化以及保存这些数据),对作业人员技术能力的要求也会相应提高。所有这些,都是在当前应对气候变化和保护生物多样性的背景下,对更好地了解环境、减少灾害的真实社会需求的回应。
环境百科全书由环境和能源百科全书协会出版 (www.a3e.fr),该协会与格勒诺布尔阿尔卑斯大学和格勒诺布尔INP有合同关系,并由法国科学院赞助。
引用这篇文章: LALLEMENT Christian (2024年3月6日), 水文测验:为什么要测量河流流量,怎么测?, 环境百科全书,咨询于 2025年1月21日 [在线ISSN 2555-0950]网址: https://www.encyclopedie-environnement.org/zh/eau-zh/hydrometry-measuring-flow-river-why-how/.
环境百科全书中的文章是根据知识共享BY-NC-SA许可条款提供的,该许可授权复制的条件是:引用来源,不作商业使用,共享相同的初始条件,并且在每次重复使用或分发时复制知识共享BY-NC-SA许可声明。