The dangerous liaisons of oxygen and life
PDF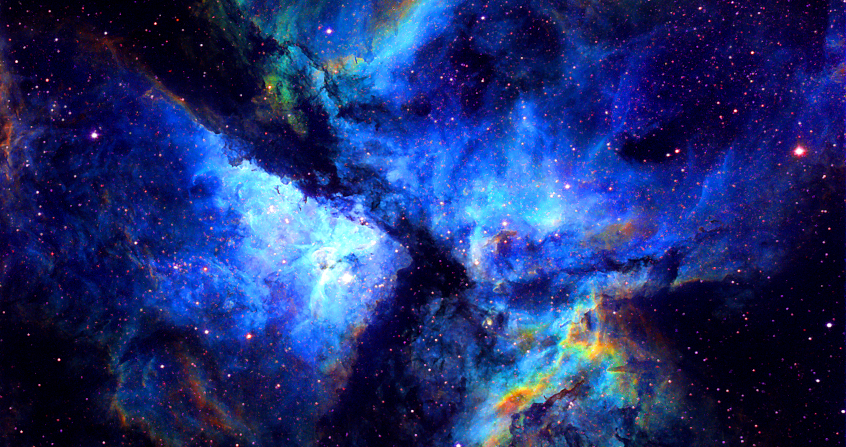
Oxygen is life! In any case today many biological processes could not take place without molecular oxygen, O2, and many organisms die when they are deprived of it. But other organisms do very well without oxygen, it is even a violent poison for some of them. And in the past? In fact, for nearly two billion years, life developed on Earth without oxygen, simply because there was little or no oxygen in the Earth’s atmosphere or in the water of the oceans (water that is absolutely essential for life!). In fact, the oxygen molecule is a by-product of the metabolism of certain bacteria, the cyanobacteria. Life created oxygen! Then it had to manage to continue its story in the presence of this powerful oxidant. It is this turbulent confrontation between life and oxygen that is discussed throughout this article.
The oxygen molecule, O2, also known as dioxygen, is very closely associated with our idea of life. Along with the water molecule, it is probably the most important molecule that characterizes life in the eyes of the general public. No life without oxygen! So much so that the possible decrease in its rate in the air that would be induced by the massive deforestation of the Amazon is presented as the harbinger of a planetary catastrophe [1]. However, there is nothing absolute about the life-oxygen link. There was a time when, on our planet, life developed and persisted in the absence of oxygen, and even today many forms of life still do very well without it. In fact, it is often a terrible poison for them. There is a good chance that if it had been abundant in the early history of our planet, life would never have appeared on it.
1. What’s oxygen?
Other molecules consisting only of oxygen atoms exist. The best known is ozone, O3, which is present in the Earth’s upper atmosphere, where it is produced from O2. Ozone effectively protects us from some of the solar ultraviolet radiation that it is able to absorb. Other infinitely rarer forms of oxygen can be obtained in the laboratory: O4, and even O8 in solid oxygen.
2. What’s the oxygen for?
The mechanisms of oxidation reactions are complex and are always associated with reduction mechanisms. We talk about redox processes. [3] In the reaction in Figure 2, glucose is oxidized (to CO2 and H2O), while oxygen is reduced (to H2O). All the reactions involved can be interpreted in terms of electron transfer. A molecule is reduced when it accepts an extra electron, it is oxidized when it loses an electron. Oxygen, O2, which is reduced to water, is therefore an electron acceptor. At the end of a chain of redox reactions, for example the respiratory chain, it is the oxygen that captures the electrons involved. It is said to be the final electron acceptor.
Humans, like all animals and fungi, use organic molecules (e.g. sugars) as electron sources and as acceptor O2: they breathe! Plants also breathe and need oxygen to live (even if they are able to produce it through photosynthesis; see Shedding light on Photosynthesis). All this makes us unconditionally associate life and oxygen. Yet other electron acceptors are possible.
3. Life without oxygen today
For many organisms that live in anaerobic conditions, oxygen is not only useless, it is also toxic. It’s a poison that destroys them quickly. For them, oxygen is synonymous with death.
4. Life without oxygen in the beginning
Four billion years ago, when life was beginning to develop, the Earth’s atmosphere was devoid of molecular oxygen. Of course, it’s difficult to be certain about the composition of the early atmosphere. At first it was thought to be largely made up of hydrogen, methane, ammonia. Then it would have been very reductive. Especially if a few oxygen molecules had formed, they would have quickly disappeared, reacting with hydrogen to give water (O2 would have been reduced). But it now seems clear that the atmosphere was mainly composed of carbon dioxide, CO2, and water vapour, a more acceptable medium for molecular oxygen to remain in the atmosphere. But the water of the terrestrial ocean contained a lot of ferrous iron (Fe2+), which is soluble in water. Any trace of oxygen would have reacted by oxidizing the ferrous iron to ferric iron (Fe3+), its most oxidized form.
It is therefore quite certain that the first life on Earth functioned anaerobically, without oxygen (see Once upon a time when life appeared: chemistry in the earth’s ocean 4 billion years ago). If we consider that life on Earth appeared about 4 billion years ago, which seems reasonable, then for more than a third of that time, oxygen was not the final electron acceptor (the oxidant) of any living form! But then what was the oxidant? We have seen that today it could be sulfates or sulfur. Nitrogen derivatives, nitrates and nitrites, can also be suitable. They’re all potential oxidizers for the origin of life.
But an electron acceptor still effective today was then omnipresent, CO2, which is therefore a serious candidate for the role of primitive oxidant. We then think back to the methanogenic archaea, such as Methanobrevibacter smithii, which pulls in our intestines. If this was the case, then methane was formed on Earth 4 billion years ago. It is hardly possible to imagine finding any trace of this original methane today. All of the molecules formed have since been recycled to form other organic products and/or give back CO2. And even if there were very small amount, how could it be distinguished from the current biological methane? Perhaps by looking elsewhere? Methane is found on Mars. The hypothesis has been put forward that it was formed at the beginning of the Red Planet’s history, when it was partly covered by ocean and life began to develop there. Analogues of methanogenic archaea on the very young Mars? We’re a long way from little green men, but we would have more plausible Martians there! Life having then disappeared, the Martian methane could have survived until today, and would thus be a fossil of archaea.
5. The history of oxygen and its influence on living things
5.1. The great oxidation: a few percent of oxygen
This considerable event, which lasted from 2.4 to 2 billion years, is called the Great Oxidation Event (GOE). [8] This “oxygen crisis” was catastrophic for a considerable number of anaerobically metabolized species. Probably most of them disappeared. This is perhaps the largest mass extinction on Earth. A mass extinction of “tiny microbes”, less telegenic than the extinction of the dinosaurs, but with huge consequences. Just as some avian dinosaurs (birds) survived the Cretaceous extinction, while all the other dinosaurs disappeared, some ancient bacteria and archaea, by taking refuge in non-oxygenated biotopes, or by adapting, have successfully passed the GEO crisis (so that prokaryotes still exist).
Among the adaptive processes, one has allowed for considerable good evolution. Before the GOE, no organism had a cell nucleus, by the end of the GOE some had. Eukaryotes were born! It is thought that they were archaea that transformed. To resist oxidative stress, they would have developed internal membranes, one of which would have become the nuclear membrane. Within this extra membrane, the DNA of the new species would have been better protected from oxygen attack. Then, or at the same time, bacteria would have come to phagocytize these proto-eucaryotes, perhaps finding protection there. These ex-archae with a nucleus and bacterial hosts (such as mitochondria), are the eukaryotes (see Symbiosis and evolution: at the origin of the eukaryotic cell). Thanks to them (and prokaryotes with aerobic metabolism), it is possible to associate life and oxygen.
5.2. And even more oxygen!
It is more than likely that the two events, the significant increase in the amount of available oxygen and the multiplication of large multicellular species, are linked. This increase is the consequence of relative variations between photosynthesis and trapping of organic matter in sediments that produce oxygen and other phenomena that consume it, such as the oxidation of iron of magmatic origin, mineral sulphur, ancient sedimentary rocks, etc.
Aerobic breathing, which consumes O2, then provided a massive supply of energy that made this explosion of life possible. Here at least, oxygen is life, even in its excessiveness. It is perhaps thanks to its abundance in the Permian (23% as opposed to 21% today), 300 million years ago, that a kind of giant dragonfly 30 centimeters long with a wingspan of 70 centimeters, Meganeura, (Figure 6) was able to develop. According to the authors who present this hypothesis, there would not be enough oxygen on earth today to allow Meganeura to live, and the largest dragonfly today has a wingspan of just 20 centimeters (which is already not bad!).
5.3. Too much oxygen?
But getting to “too much” oxygen can lead to serious problems, starting with ice ages. As long as there wasn’t too much, methane would remain in the atmosphere, and methane is a powerful greenhouse gas. It maintained a climate that could be described as “globally tropical” on our planet. But the increase in oxygen levels led to its oxidation into CO2. CO2 (we talk a lot about it, and rightly so, with the current global warming) is also a greenhouse gas, but much less than methane (more than 20 times less per unit of mass). Replacing methane with carbon dioxide therefore means cooling the Earth, so that gigantic glaciations took place at a time aptly named the Cryogenian (from the ancient Greek krùos, cold), 700 to 600 million years ago.
It is even conceivable that the entire surface of the planet has been frozen, creating a “snowball Earth“. It was after this period, during the Ediacaran era, that multicellular life really took hold (see The first complex ecosystems). The fauna of the Ediacaran was extremely rich and diverse, yet it completely disappeared 545 million years ago, replaced by a new fauna, the Cambrian fauna, which is the origin of all the animals we know today. It is likely that the increased presence of oxygen in the surface water of the oceans allowed the Ediacaran fauna to explode. Its sudden disappearance may have been caused by a destabilization of the ocean water layers, leading to the upwelling of a powerful toxic gas, hydrogen sulphide H2S.
Sixty-five million years ago, when a gigantic meteorite hit the Yucatan Peninsula in Mexico, causing the disappearance of all non-avian dinosaurs (see Massive extinctions in geological time), oxygen was a few percent more abundant than it is today. These few percent must have had significant consequences. The first dinosaurs (and other animals) to disappear were those of the Yucatan. Then the shock wave reached North and South America, the front of the wave spreading in a huge circle, and crushing everything in its path. A huge Tsunami completed the picture and drowned everything that was not high enough above sea level as far as Europe and Africa.
So far, the excess oxygen had nothing to do with it. But then… glowing ash rained down on the entire planet. They started millions of fires that soon joined together to form one on the scale of the entire Earth. And that’s when the few percent extra oxygen played a nasty trick on the dinosaurs. Many of them lived in wetlands. With 21% oxygen as it is today, some forests might not have burned. In any case, there would have been some mangroves, some swampy heaths that wouldn’t have burned. And a number, perhaps small compared to their initial population, but not negligible, of dinosaurs would have passed this stage. Perhaps enough for some to successfully face the climatic cooling that followed, caused by the impenetrable layer of smoke that prevented the sun’s rays from reaching the ground. Dinosaurs have been known to live in areas with harsh winters. So why not? But, definitely, too much oxygen is dangerous.
6. A precarious balance
As we’ve seen, indiscriminately associating life and oxygen is a mistake. There has been and still is (even inside human beings) life without oxygen. For a third of its history, life on Earth has fared very well without it. It even feared it, to the point of a serious crisis when cyanobacteria produced it en masse in the oceans and in the atmosphere. Fortunately, the great oxidation was a slow enough event (several hundred million years) for bacteria and archaea to adapt and survive this kind of first world chemical warfare. Evolution allowed the selection of archaea that had a nucleus and associated with bacteria (strength in numbers!). Eukaryotes were born. They will take a long time to become animals and plants. This time oxygen will help them do so. But it’s never far away from the point where that same oxygen could burn all the organisms on Earth.
Terrestrial oxygen is taken up in a vast geochemical cycle, linked to the cycle of other elements, mainly carbon, via the respiration of living beings, their decomposition, their burial, the formation of carbonates (layers of limestone) or that of hydrocarbon and coal deposits. It is also linked to the sulphur cycle, by forming sulphates. The stability of the oxygen level is therefore the result of numerous chemical equilibriums, but also of physical processes. If all the organic matter produced in the oceans was oxidized, they would not release any. In fact, it is because a very small part of this organic mass is buried before being oxidized that the balance remains positive. The equilibrium is very precarious and is played out to almost nothing: to within 0.0001%! [9]
7. Messages to remember
- It is essentially geochemical and geological phenomena that determine the amount of oxygen, O2, present in the atmosphere.
- For more than a billion years, life developed on Earth in the absence of oxygen.
- A little over two billion years ago, the appearance of oxygen, the great oxidation, was a considerable event, which turned life on Earth upside down. It was then that eukaryotic cells (with a nucleus) appeared.
- It was when the oxygen level increased again to reach 20 to 30% that pluricellular life, as we know it today, appeared and diversified (animals, fungi, plants).
- Oxygen is essential to life today, but it is a powerful oxidant. Too much oxygen could be detrimental to its maintenance, or in any case, modify it very seriously.
Notes and References
Cover image. Image of oxygen in the Great Carina Nebula. [Source: Original photo by Dylan O’Donnell, deography.com; derivative work by Tobias Frei / CC0]
[1] Zimmer K., Why the Amazon doesn’t really produce 20% of the world’s oxygen ? National Geographic, 28 Août 2019
[3] Réaction d’oxydo-réduction : un transfert d’électron (La chimie.net)
[4] Les archées : rencontre du troisième type (MNHN).
[5] Boisson T., Feux follets : que sont en réalité ces étranges lumières ? Trust my Science, 2018,
[6] Gootlieb K., Wacher V., Sliman J. & Pimentel M., AP&T, 2016, 43, 197, DOI : 10.1111/apt.13469
[7] Claire König. Les cyanobactéries : apparition, adaptation et reproduction. (Futura Sciences)
[8] The event that transformed Earth (BBC).
[9] Field C.B., Behrenfeld M.J., Randerson J.T. & Falkowski P., Primary Production of the Biosphere: Integrating Terrestrial and Oceanic Components. Science, 1998, 281:237-240. DOI: 10.1126/science.281.5374.237
The Encyclopedia of the Environment by the Association des Encyclopédies de l'Environnement et de l'Énergie (www.a3e.fr), contractually linked to the University of Grenoble Alpes and Grenoble INP, and sponsored by the French Academy of Sciences.
To cite this article: LEQRAA Naoual, VALLÉE Yannick (July 22, 2020), The dangerous liaisons of oxygen and life, Encyclopedia of the Environment, Accessed November 21, 2024 [online ISSN 2555-0950] url : https://www.encyclopedie-environnement.org/en/life/the-dangerous-liaisons-of-oxygen-and-life/.
The articles in the Encyclopedia of the Environment are made available under the terms of the Creative Commons BY-NC-SA license, which authorizes reproduction subject to: citing the source, not making commercial use of them, sharing identical initial conditions, reproducing at each reuse or distribution the mention of this Creative Commons BY-NC-SA license.