植物对水孜孜不倦的追求
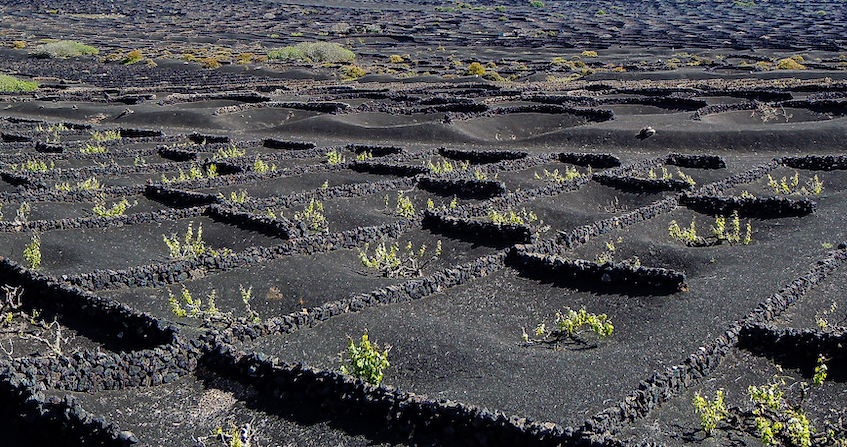
水是如何到达树木叶片的?植物用什么方法来应对缺水的环境?植被是如何改变气候的?生命起源于水中,但陆生植物却不得不适应暂时性的水资源短缺。植物的根可以从土壤中吸取水分,叶片通过蒸腾作用施加一个吸力,将水分通过茎干吸取上来。因此,水在茎干中会处于张力或负压状态下。当这种张力由于缺水变得过强时,管腔内会产生气泡导致空穴,使水分无法继续传输。鉴于蒸腾作用是由气孔调节的,为了避免这种情况,这时气孔就会关闭。但气孔关闭的同时也限制了CO2进入叶片,从而限制了光合作用和生产。
1. 植物和水:主要原理
1.1. 植物需要液态水
对于家里种植有几株植物的人来说,不用说也知道植物必须要定期浇水,有些需要经常浇水,有些则需要少浇。所有生物都是由充满水分的细胞组成的,植物的含水量通常在70%-95%之间。当然也有一些例外:树木木材的含水量仅占30-70%,种子几乎可以完全干透,但仍能够发芽。
1.2. 植物也需要空气,在饥饿与干渴之间权衡
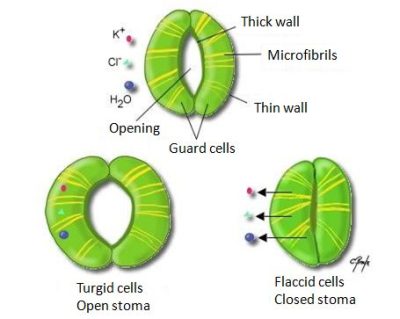
一个人可以几个星期不进食,但只能几天不饮水,但是人可以主动去寻找水或食物。但植物无法移动(参见“植物固定的生活及其限制”),它吸收二氧化碳(CO2),空气中CO2的浓度很低(按体积算为400ppm或百万分之一)。为了吸收这些CO2,植物需要与外界空气接触(参见“光合作用中的碳途径”)。植物发育形成了内外部交换面,外部通过茎叶、内部通过大面积的细胞与空气接触。但与外界接触会导致水分流失:植物细胞中的水分会蒸发,而且水蒸气通过叶和茎的表皮(或皮肤)逸出,这就是蒸腾作用。人体中,蒸腾作用是先排出液态水后再进行蒸发。植物中,叶片内部的空气中发生了蒸发,而后水蒸气通过气孔散发到大气中。气孔(stomata)一词源于希腊语stoma(口),因为stoma像有两瓣唇(两个细胞)的嘴,可以打开或关闭(图1)。
当植物缺水时,它会关闭气孔限制水分流失,但这也阻止了CO2的吸收,增加了饥饿的风险。因此,在保证生存的同时,植物必须不断地调节气孔的张合,以调节水分的流失。气孔至少在400百万年前就已经存在,而植物325 百万年前就优化了与外界气体交换的能力。
1.3. 植物体内的水分是处于压力还是张力之下?
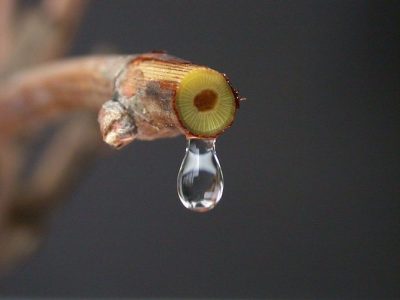
细心的观察者会被植物在矿物环境中开疆拓土的能力所震惊。树根可以抬起路面的水泥板,绿芽可以穿透墙壁,或者穿过不太繁忙的道路。正是根茎中的水压使得这些活动得以进行。因此,植物中的水分有时是会受到压力的,长叶前茎被切断时,水分就会流出来。春天修剪时“哭泣”的葡萄藤蔓正是这种情况(图2),可以收集树液的桦树也是如此。[1]
这种水压在植物细胞中永久存在,能推动细胞壁生长,使细胞膨胀、增加植物器官的体积和的大小,从而促进植物生长。
但当树木有叶子时,就不能收集汁液了,因为白天时树木会发生蒸腾作用,茎干中的水处于张力下,这是一种由于树叶蒸腾而产生的负压。这是植物中最常见的情况,我们后续阐述。
1.4. 水在植物中是如何循环的?
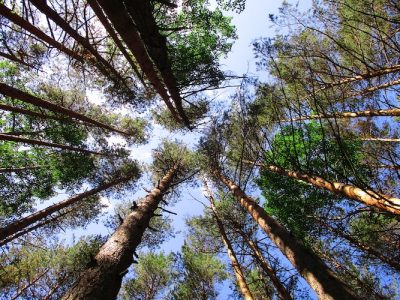
日常生活中,水是在重力作用下循环的,也就是地心引力的作用。我们能从水龙头中获得自来水,因为自来水来自建得高处的水塔。当然,需用水泵将水从下方的水库中注入水塔。这些泵建在水库中,产生压力将水推入水塔中。而如果想从井里打水,可以使用水桶或水泵。如果是浅井,可以在地面使用吸水泵。它能产生相对真空,大气压将井水推入这个真空,把水抽到地面。但是吸水泵不能产生负压,只能把水抽到最高10米处,此时的压力相当于一个标准大气压。
然而,我们周围15米至30米高的树都能顺利地为叶子提供水分(图3),甚至加利福尼亚和澳大利亚有高达100米的树。它们是怎么做到的?长期以来,这个问题长期以来一直存在争议,因为答案有悖常理,但现在已经没有疑问了。
存在于叶片细胞内和细胞间隙中的水分,白天会在叶片内部蒸发。这种水分的流失会对导致蒸发部位的液态水产生吸力或产生负压。由于水分子在静电作用下紧密结合在一起,这种负压会将水通过根茎从土壤中吸到叶片里。这样,植物可以从10米以上的高度吸水,这是人类用吸水泵无法做到的。
我们已经知道,水在重力和压力差的作用下循环。水中溶解的盐分也通过渗透力吸引水分子。当水处于土壤或细胞壁等多孔介质中时,会通过毛细作用与固体基质结合。物理学家试图把这些不同的力统一为水势或水势能的概念,因为水总是沿着势能减小的方向流动。为了进一步阐明这一点,我们需要更详细地了解水的物理性质、水势的概念和蒸腾的影响因素。
2. 植物和水:细节部分
2.1. 水的物理性质
水分子是一个由两个相互形成104°角的O-H键构成的电偶极子,该分子结构使得水具有显著的性质(图4)。这个偶极子的正极吸引另一个分子的负极,以保持了液体的凝聚。这种内聚力很重要,解释了蒸发水所需的高能量:平均590cal/g,或2450J/g或2.45 MJ/kg。这种能量是所有液体中最高的,大约是将水从0°C加热到100°C所需能量的6倍。
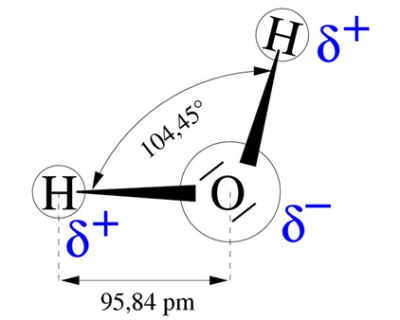
因此,蒸发具有很强的冷却能力。夏季干燥的沥青表面所测温度为49°C,而附近池塘的表面温度是26°C,潮湿草地或桤木表面为29°C。植物尤其是树木在调节气候的过程中发挥着重要作用。由于深根,树木在整个生长季节都能保持郁郁葱葱,这也使得它们在旱季仍能保持水分的供应。
水分子间的强内聚力也使液态水具有高密度,且水比冰的密度高,因而冰能够漂浮在水面上。水的表面张力很高,这使得水黾(被误称为水蜘蛛的昆虫)能在池塘表面奔跑。
2.2. 水势及其组成
水势Ψ是水的势能,受重力、渗透势和基质势以及压力差的影响而变化(参见“物如何应对盐胁迫?”)。它用焦耳/立方米(J m-3)表示,这是压力(帕斯卡,Pa)的一种测定维度。Pa是一个非常小的单位,所以常使用MPa或bar(1bar = 0.1 MPa)。
重力势Ψg为每单位体积V的重力势能m.g.h,其中m为水的质量,g为重力加速度,h为到参考面(一般为地面)的高度:
-
-
- Ψg=(m/V).g.h=ρ.g.h
-
如果水在地面以上(h>0),则Ψg为正值,否则是负值(h<0)。因此,在一棵树中,根部水分的Ψg值为负,叶片水分的Ψg为正值。
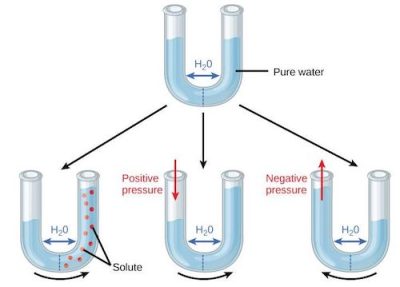
渗透势或溶质势Ψs为水与溶质结合产生的势能。如图5所示,使用由半透膜隔开的U型管,半透膜可透水但不透溶质。在最上方的U形管中,纯水可在管道中自由穿过半透膜,使得两侧水位相同。图左下方,一种溶质(红点)被放置在右半室,左半室的纯水被盐分子吸引,水就会上升到右半室。在图的下部中央,对左半室的水施加正压模拟了同样的运动,而在图右下方,如果对左半室的水施加负压,运动方向则相反。
渗透势Ψs始终为负值,因为与溶质结合的水比纯水更少。渗透势取决于溶质的浓度Cs。对于稀释溶液来说,这种相关性大致上是线性的:
-
-
- Ψg=-R.T.Cs
-
R是理想气体常数,T是绝对温度
基质势(Ψm)是将水与土壤或细胞壁等基质中的固体颗粒相结合的能量,它与接触面(球形孔隙接触面为4πR2)成正比,与体积(4πR3/3)成正比,方差为1/R,即孔隙半径的倒数。基质势总是负值,尤其是当孔隙很小的时候。因此,孔隙较大的砂质土保水量很小,而孔隙小的粘质土保水量则大得多。植物可利用的土壤水分在两个限度之间变化:
- 上限:田间持水量;
- 下限:永久萎蔫点。
水分含量高于田间持水量时,水在重力作用下流动;低于永久萎蔫点时,植物无法利用残余水分,相当于Ψm=-15bar(或-1.5MPa)。
介于这两个限度之间的水分含量称为有效含水量,对于1米深的土壤而言,有效含水量指100至200毫米的水位,这代表在良好条件下作物30至60天的水分消耗量。实际上,当储水量一半被消耗殆尽时,植物的气孔就会关闭。如果根深小于1米时,储水量会更低。但树木的根要远深于1米,因此增加了有效含水量。巴黎萨克雷大学植物生态生理学实验室的研究人员称,梅伦附近的巴尔博无柄栎林的有效含水量可以达到400毫米水位(见下文)。
最后,压力势ΨP与压力相等,因此白天,膨胀的细胞中ΨP为正值,而导管中ΨP为负值。
因此水势Ψ是这四个分量的总和,水总是沿着水势降低的方向流动:
Ψ=Ψg+Ψs+Ψm+ΨP
图6显示了白天树木蒸腾作用时水势的变化:
土壤水势略为负(-0.05MPa或-0.5bar),因为地表以下的水,含有低浓度的溶解性矿盐。图6. 树木中水分根据水势递减而流动的循环图。[图片来源:作者自制]( Mesophyll cells 叶肉细胞,Xylem 木质部,Stoma 气孔,Transpiration draws water from the leaf 叶片通过蒸腾作用丧失水分,Cohension and adhesion draw water up the xylem 在木质部内,水分子的内聚力和粘附力促使其上行,Root hairs 根毛,Soil particle 土壤颗粒,Water molecule 水分子,Negative water potential draws water into the root 负水势使得水被吸入根中,Low 低,High 高,Atmosphere 大气,Leaf at top of tree 树顶端的叶片,Stem 茎干,Root cells 根细胞) - 在树顶,叶片的水势是-1.5MPa或-15bar,因为叶片有大量蒸腾作用。如此大的负值使得从根部到叶片的整个水柱产生吸力,使根(-0.2 MPa)和树干(-0.6 MPa)的水势处于中间值。由于水分子之间的内聚力,水柱能够承受负的水势(和由此产生的压力)。
- 注意,大气水势(- 100mpa)远低于叶片的水势(-1.5 MPa)。大气水势与空气的相对湿度平衡,在这种情况下,露天空气的相对湿度为 48%,而叶片内空气的相对湿度为9%,因此叶片内的空气几乎处于饱和状态[2]。
树的最大高度约为100米,此时重力势相当于10巴或1MPa。这意味着叶片的水势必须远低于-1MPa,才能将水输送到这个高度。
2.3. 蒸发、边界层和气动阻力
水以蒸气的形式存在于空气中。当水蒸气遇到干燥的空气时,水蒸气压力值(e)会增加到最大值,超过该值,水蒸气会凝结成液态水。这称为饱和蒸气压es(T),它随温度呈指数增长(图7)。水蒸气压力(e)/饱和蒸气压es(T)的比值为相对湿度,用%表示。饱和蒸气压es(T)与水蒸气压力(e)之差为水分饱和差,会随温度升高而急剧增加。
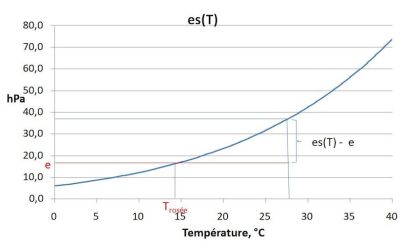
在叶片内部,水蒸气实际上已经饱和,叶片的蒸腾作用是由于叶片内外部的蒸气压力差。
与液态水接触的空气中水蒸气是饱和的,自由水面的蒸发量E取决于水面与自由空气间的水分饱和亏差[es(T)-e]。E随风速的增加而增加,我们将此定义为气动阻力ra,即水气在蒸发面和自由空气之间流动时,空气所施加的阻力E。
大多数情况下这种阻力位于边界层,边界层指从空气速度为零的表面延伸到空气中的区域。叶片的边界层厚度约为一毫米,开阔水面的边界层厚度为几厘米,玉米地的边界层厚度可达几十厘米,森林的边界层厚度可达几米。
2.4. 蒸腾作用
气动阻力ra适用于池塘,湖泊等开阔水面或潮湿的物体,如潮湿的吸水纸。对于植物来说,水蒸气必须首先通过表皮和气孔扩散,然后进入到大气中,这个阻力用rs表示,再加上ra就是两个连续的阻力。
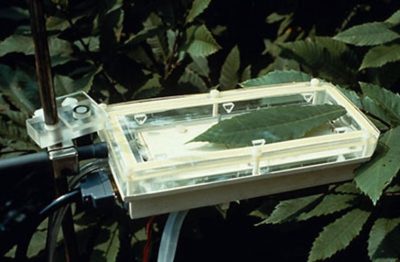
在田间,可以用封闭箱体将叶片封闭(图8)或将枝条上的所有叶片全部封闭(图9)来测量来叶片的蒸腾作用和气孔阻力。[3]
叶片通常被比作吸水纸,在物理因素的影响下可以自由蒸发。太阳辐射提供了大部分蒸发的能量,风速改变阻力ra,空气温度和湿度影响空气饱和蒸气压差。辐射水平越高,空气越热、越干燥,蒸发量就越大。叶片和吸水纸的区别在于叶片的表皮和气孔减缓了蒸发,类似一张有小孔的塑料片:
- 气孔完全张开时,叶片的蒸腾作用接近吸水纸的蒸腾作用(但略小);
- 当气孔关闭时,由于表皮角质层残留的孔隙,蒸腾作用会降到非常低的值。这就导致了叶片不再通过蒸发进行冷却,叶片的温度升高。
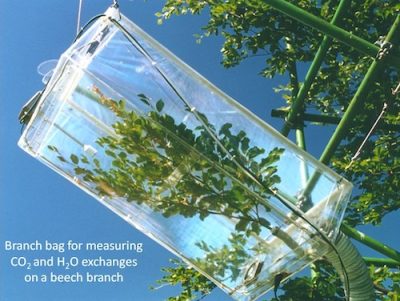
因此,蒸腾作用取决于影响蒸发的物理因素,也取决于称为气孔阻力的生物变量,气孔阻力是纯物理气动阻力的补充。这种阻力取决于气孔的密度和大小,可以用物理模型进行模拟和计算。
2.5. 蒸散量
植物冠层上的通量E被称为蒸散量,是土壤和湿润表面(如有)的蒸发量和叶片蒸腾量的总和。它与叶面积指数密切相关,叶面积指数是植被叶面积与下层土壤面积的比值。
实际上,自然地表蒸发的水分量取决于地表条件(开阔的水面、不同的植被覆盖度和土壤湿度)和气候条件(太阳辐射、空气温度和湿度、风速)。
潜在蒸散量Ep是完全覆盖于地表并有充足水分供应的低矮植被表面蒸发的水量。Ep随气候变化从0到10毫米/天不等;在像萨赫勒这样炎热干燥的地方,在一年多的时间里,绿化良好的灌溉草地,Ep最大可以达到2700毫米。
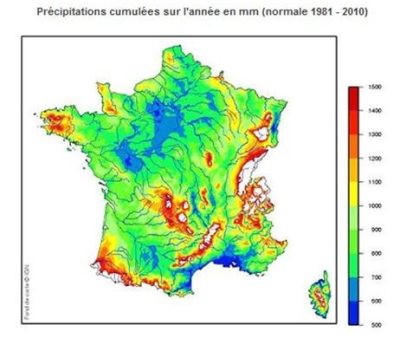
实际蒸散量显然要低得多,因为植被并不总是覆盖地表,而且常常受土壤水分的限制。所有流入大陆的水量大致已知,平均为每年758毫米。蒸散E就消耗了其中的三分之二,即490毫米/年,其余的则流走汇入了河流和溪流。这个数字是E值极低的表面(南极和格陵兰冰区和干旱地区,占大陆总面积的41%)和其他高E值地表(如热带雨林,平均降水量为1300毫米/年)之间的平均值。法国的平均降水量为932毫米/年(从500毫米/年到1500毫米/年不等,具体见图10)和蒸散量为577毫米/年,相差的355mm/年形成径流。如果土壤不缺水,法国北部的茂密森林或长势良好的作物在夏季每天会损失3到4毫米的水分,而在地中海区域,水分损失会更多(4到7毫米)。
利用传感器测量植被上方的风速、温度和空气湿度的局部波动,可以持续评估实际蒸散量。巴黎萨克雷大学植物生态生理学实验室自2005年以来就一直对枫丹白露附近的橡树林进行此类测量图11)[4]。
世界上有数百个这样的设备,2010年首次绘制了蒸散量通量变化图(图12)[4]。赤道附近热带雨林地区的蒸散量最大(高达1300毫米/年),干燥的热带地区蒸散量减少,然后向温带地区增加,最后又由于温度和辐射的降低而向两极递减。
3. 水在植物生产中的作用
3.1. 植物是否需要蒸腾作用?
当然,因为叶片需要与外界的空气接触才能吸收赖以生存的二氧化碳,这就是为什么叶片表面有许多气孔(参见“空气污染物对植被有什么影响?”)。叶片内部的空气非常潮湿,因为它与水分充足的细胞接触,植物或动物细胞不能在缺水的环境下存活。叶表皮有多个气孔,以及叶片内外空气之间的湿度差导致了蒸腾作用。除了碳元素,植物还需要其它矿物质元素(氮、磷、钾等等),这些元素存在于土壤溶液中,通过蒸腾作用被根系吸收。
据估计,生产一公斤小麦,作物需要消耗大约500升水。这个数字代表的是一个数量级;由于蒸散量的变化,在湿润气候下耗水量略低,干旱气候下耗水量较高。
3.2. 对树液循环的影响
液态水由极性分子组成,这些分子以特别高的内聚力相互吸引。高内聚力使得树液可以向上流动,大树茎干中的水处于非常低的负压下。然而,当张力过大时,茎干导管中的水柱会中断并出现气泡,阻碍水的循环。这就是气穴现象,也就是导管栓塞。一棵树中有很多的导管,当气穴冲击到很大一部分导管时,树体就会开始受损[6]。导管的解剖结构及其敏感性已经得到了深入的研究。
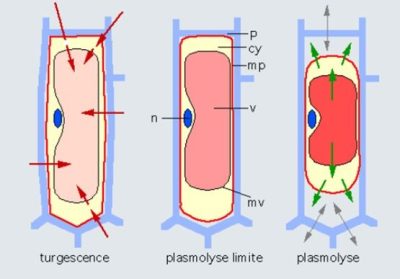
液态水也是一种很好的溶剂,在每个矿物离子周围都有一层水膜。溶质分子和水分子之间的作用力称为渗透力,溶液浓度越高,吸引的水就越多,从而产生浮力(在希腊语中,渗透力即指浮力)。
构成植物的细胞中有水,从根到叶为植物提供养分的导管中也有水。土壤中的水通常矿质元素含量较低,而在细胞中的水则相当浓缩。这种浓度差异使得水通过渗透作用从土壤流到植物体内,使细胞膨胀(图13,左图)。因此,细胞内的水通常处于压力下,此时细胞也被称为是膨胀细胞。这种压力被细胞壁所缓冲,细胞壁是由相对坚硬的纤维素和果胶组成的,其作用就像一个承受内胎压力的轮胎。汽车轮胎的气压为2到3个大气压,自行车车胎的气压可达6个大气压,植物细胞的膨压可高达10个大气压。正是由于这些高压,植物细胞才需要细胞壁,而动物细胞则没有细胞壁,因为动物细胞承受的膨压较低。当细胞变干燥时,这种压力就会降低,然后随着细胞膜与细胞壁分离,这种压力彻底消失,这就是所谓的质壁分离(图13,中和右图)。
4. 总结
- 水是所有生命形式所必需的介质。
- 植物细胞中的水处于压力之下,这种压力促使植物生长,并确保植物具备一定的形状和硬度,以及使得茎和树干出现木质纤维素组织。
- 另一方面,水在叶片和导管中处于张力状态,这种张力使得树液上升,弥补了叶片蒸腾和蒸发过程流失的水分。
- 蒸腾作用主要通过气孔进行,气孔是植物吸收二氧化碳的必要条件。
- 气孔在土壤潮湿时张开,在土壤干燥时关闭,以保存内部水分,因此抑制了CO2的进入,也抑制了光合作用和生长。
- 水势的下降使气孔关闭,当水势变强时,也会通过气穴现象在导管中形成气泡。
植物对缺水的适应机制将在相关的文章(即将发表)中进行讨论。
参考资料及说明
封面照片:兰萨罗特岛(加那利群岛)拉格里亚公园的葡萄园。在低矮的石墙里,这里有着罕见的降雨量(170毫米/年),并提供着优质的葡萄酒。[来源:Patrice78500,CC BY-SA 4.0,通过维基共享资源平台]
[1] https://fr.wikipedia.org/wiki/Eau_de_bouleau
[2] 水势与大气湿度的关系式为:Ψ = (RT/Vwater)*ln(e/es(T)),其中R是理想气体常数,T为绝对温度,Veau为一摩尔液态水的体积,e/es(T)为相对湿度。假设R = 8.3 J mol-1 K-1,T = 298 K,Veau = 18.10-6 m3 mol-1,e/es(T) = 0.48,则 Ψ = – 100 MPa。因此,大气水势通常很低,除非空气和叶片内的空气一样几乎饱和(hr>98%)。
[3] Dufrêne E, Pontailler J-Y and Saugier B, 1993. A branch bag technique for simultaneous CO2 enrichment and assimilation measurements on beech (Fagus sylvatica L.) Plant, Cell and Environment, 16, 1131-1138
[4] http://www.barbeau.universite-paris-saclay.fr/index-fr.html
Jung M et al. , 2010. Recent decline in the global land evapotranspiration trend due to limited moisture supply. Nature 467, 951-954.
[5] 感兴趣的读者将在网站“植物与水:II.7.植物的水力结构、空化和栓塞”中的安德烈·格拉尼埃(André Granier)和埃尔维·科哈德(Hervé Cochard)的精彩演讲(法语)中找到更多信息。
环境百科全书由环境和能源百科全书协会出版 (www.a3e.fr),该协会与格勒诺布尔阿尔卑斯大学和格勒诺布尔INP有合同关系,并由法国科学院赞助。
引用这篇文章: SAUGIER Bernard (2024年3月14日), 植物对水孜孜不倦的追求, 环境百科全书,咨询于 2024年7月3日 [在线ISSN 2555-0950]网址: https://www.encyclopedie-environnement.org/zh/vivant-zh/tireless-quest-for-water-by-plants/.
环境百科全书中的文章是根据知识共享BY-NC-SA许可条款提供的,该许可授权复制的条件是:引用来源,不作商业使用,共享相同的初始条件,并且在每次重复使用或分发时复制知识共享BY-NC-SA许可声明。